Implications of Exosomes
As the newest field in regenerative medicine, exosome therapy is often the least understood by doctors new to the field. While still not widely used by many doctors, this is simply because it is new. However, many scientists are eager to harness the benefits of this new treatment and apply them to target various conditions that modern medicine has struggled to address.
Extracellular Vesicles and Exosomes
The discovery of extracellular vesicles (EV), small bubbles composed of protein freely among cells, has been considered one of the greatest advancements in the field of medicine. They are broadly defined as entities that exist outside of the cells of the human body and can be quite unique. Among these are exosomes, which have shown almost miraculous promise in treating inflammation, immunomodulation, cell-cell communication, and much more. Although not a cure-all, exosomes are effective in their roles, which include the communication and rejuvenation of all cells in the body, despite not actually being cells themselves.
Exosomes are created by growing a healthy stem cell culture and then disposing of the cells, leaving behind millions of exosomes created in the process. Due to their microscopic size, what is left behind must be ultra-centrifuged to concentrate them enough for patient use. Despite all their abilities, the makeup of exosomes is quite simple, consisting of amino acids and RNA. This means that, aside from their immunomodulatory properties, exosomes can be applied to a patient without causing an immune system reaction, a common concern with other treatments.
An Overview of Exosome Therapy
Generally defined, exosome therapy is the use of exosomes in a clinical setting, a new step forward that is being happily taken by physicians worldwide. Exosome therapy has rapidly become one of the most popular fields of regenerative medicine treatment globally. Painstaking research has highlighted the practical uses of exosomes, and how they can treat conditions including Lyme disease, hair loss, and arthritis. While stem cells are responsible for the rejuvenation of older cells, they might not be able to supply the information required for healing to take place—this is where exosomes come in.
Consider exosomes the traffic conductors of regenerative medicine. They stimulate attention to damaged areas and work to control inflammation. Many researchers believe that supporting the function of exosomes in the body could lead to better outcomes for patients. These scientific breakthroughs have fundamentally changed the healing process as we know it.
How Exosome Therapy Works
Exosome therapy is minimally invasive and most commonly used in patients with orthopedic injuries, but it also has great applications in anti-aging medicine and the treatment of inflammatory and degenerative diseases. Exosome treatments generally contain a combination of growth factors, messenger RNA, micro RNA, and cytokines. The resulting exosome treatment is used in conjunction with stem cell therapies to boost the healing process in patients. These exosome therapies can be administered directly into the affected area or through an IV drip for those seeking the anti-aging properties of exosomes.
Exosome injections are administered similarly to other cellular therapies—directly into the part of the body affected by disease. Patient dosing varies based on the condition and treatment plan, allowing physicians to customize them to a patient’s needs.
What Can Exosomes Treat?
As the field of regenerative medicine constantly grows, more treatments are discovered every month. Researchers have found exosomes to be an exciting alternative for a wide variety of illnesses, including arthritis therapy, where they play a significant role in bone and cartilage remodeling. Exosome therapy currently shows promise in treating the following conditions:
Hair Loss
Exosomes can regrow hair due to their growth factor content. When injected, exosomes trigger healing and the natural regeneration of hair follicles.
Lyme Disease
Exosomes may benefit patients suffering from Lyme disease, a complex disease that compromises the immune system. By incorporating exosomes into a treatment plan, patients could break the cycle of inflammatory response.
Arthritis
Arthritis, characterized by joint inflammation due to autoimmune deficiency, can be alleviated with exosome therapy. Although various treatments are available, exosomes show potential in reversing the disease’s progression.
Anti-Aging
Aging can be thought of as a slow, steady inflammation of the body. Researchers have found a direct correlation between the number and types of exosomes within the body and the effects of aging. Exosome therapy could reduce swelling and treat conditions like crow’s feet, puffy eyes, and other markers of aging.
Pain Management
Many pain symptoms result from inflammation. For severe, chronic conditions that cannot be treated with over-the-counter options, exosome therapy could be key. By targeting the pained area at the source of inflammation, exosome therapy can increase the quality of movement and reduce pain in certain orthopedic conditions, including stress injuries.
As physicians around the world continue to study and apply exosomes, Global Stem Cells Group recognizes the need to get these products into doctors’ hands. Our Cellgenic line has been in operation for years, helping physicians treat these conditions and more with exosome therapy.
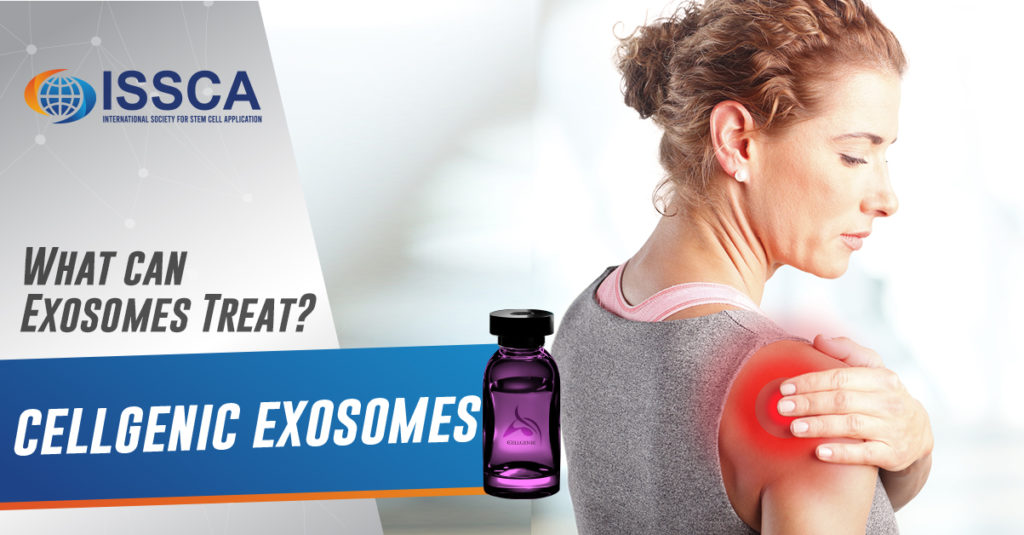
- Published in Corporate News / Blog
GCell: The Newest All-In-One Solution in Regenerative Medicine
Are you a physician currently utilizing adipose tissue but growing tired of the time-consuming, arduous procedure? Are you having problems finding reagents that allow you to isolate stem cells? If so, GCell might be the solution for you.
What is GCell?
GCell is a revolutionary new machine poised to become the future of adipose stem cell processing. Its compactness and short processing time make it a valuable implement in any regenerative medicine practitioner’s office. GCell employs a process of mechanical breakdown, which shortens the treatment duration and keeps it within legal minimal-manipulation regulations.
How Does GCell Work?
Through a system of extremely small blades and filters, GCell homogenizes a sample of fat taken from anywhere in the body, turning it into a slurry of growth factors, proteins, and other components. GCell then filters out the stromal vascular fraction cells and uses a process called photoactivation to increase their vitality. The end result is a final product that can be administered to patients within forty minutes from the sample’s harvesting. This is a significant improvement over the previous, multi-hour long treatments that physicians have grown accustomed to, and this shortened timespan is greatly appreciated by both doctors and patients.
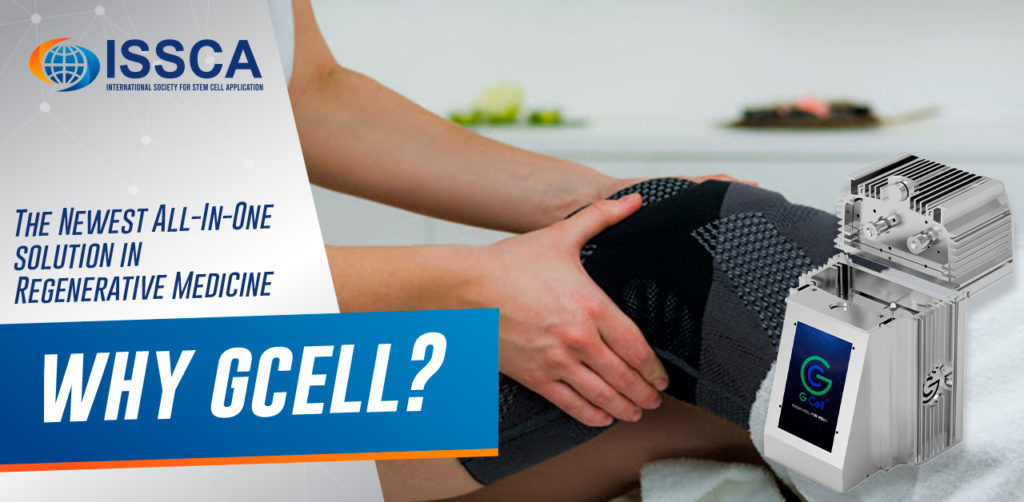
Why GCell?
GCell offers a single-session treatment, meaning patients only need to visit their clinic once without requiring follow-up treatments. This machine combines the convenience of its small size and lightweight build with the ability to accelerate the stem cell harvesting process. Additionally, GCell is designed to be an entirely enclosed system, ensuring samples remain in a sterile environment, which preserves the viability of samples and ensures positive outcomes for patients.
GCell: The Newest All-In-One Solution in Regenerative Medicine
GCell combines a grinder, a filter, and a photoactivation device, offering a turnkey solution that can take a physician from harvesting a fat sample to administering an injectable regenerative medicine therapy in less than an hour. GCell is especially useful for clinics just beginning to implement regenerative medicine into their practice, with its all-inclusive approach to stem cell isolation setting it a cut above the rest.
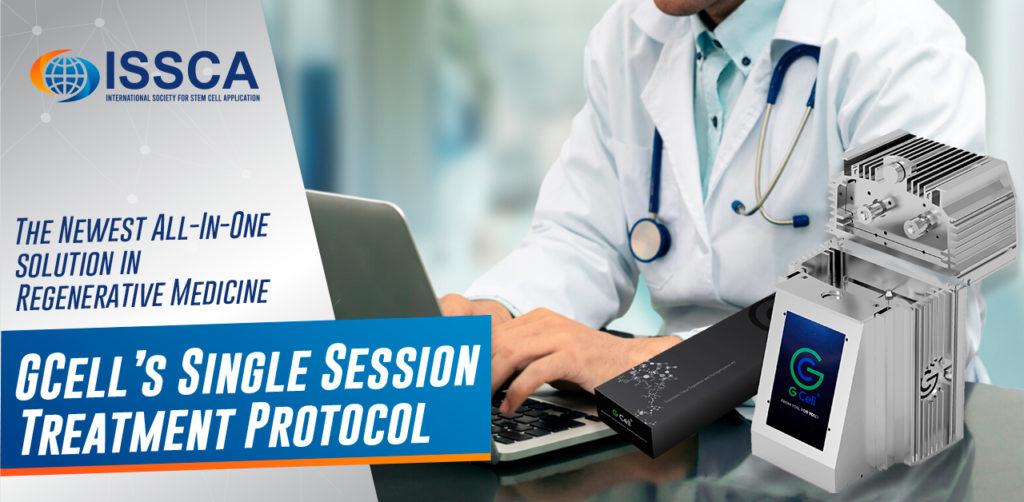
GCell’s Single Session Treatment Protocol
Unlike many other cellular therapies on the market, GCell provides a single-session treatment, eliminating the need for patients to return after their original treatment day. Its portability, small stature, and ability to accelerate the stem cell harvesting process make it ideal for clinics new to regenerative medicine, taking much of the rigor out of adipose-derived stem cell harvesting.
- Published in Corporate News / Blog
How to Boost Your Immune System to Fight Cancer
The human immune system helps to protect the body against illness and infection caused by bacteria, viruses, fungi, and parasites. This process is achieved by a collection of reactions and responses made by the body to damage these infected cells. It is also called immune responses.
This immune system is very crucial in cancer patients to help fight cancer. It identifies cancer as abnormal and destroys it, but this is not enough to rid the body of cancer altogether. In most cases, cancer will weaken this immune system because it spreads into the bone marrow that produces blood cells that fight against infections and cause the bone marrow to decrease its blood cell production.
When the immune system is unable to recognize these cells as abnormal, cellular growth will occur, causing uncontrolled growth and division of cells, leading to cancer.
The Importance of a Strong Immune System in Cancer
Cancer has been a serious and sensitive topic for both patients and doctors. Being diagnosed with cancer is frightening and tormenting, but due to the latest treatment methods, recovering from this disease is increasingly possible. Living with cancer often brings an initial psychological crisis, but surprisingly it affects not only you but also your family and others close to you.
Cancers, especially genetic cancer, can’t be prevented, so it is essential to be proactive about your health. If you’re diagnosed with cancer, some individual lifestyles will help improve your cancer care, which includes managing stress. Reducing your stress level can help you to maintain physical and mental health; you can use relaxation techniques such as meditation and yoga. Getting enough sleep is also crucial for patients living with cancer; this will help improve their health, mood, and coping ability. Also, it is best to limit exposure to toxins that can increase one’s risk of being exposed to other deadly diseases.
Healthy Feeding Habits
A healthy feeding habit is critically important and can help manage cancer side effects, improve health, and quick recovery. Some tips to help you develop a good and healthy diet include:
- Consuming assorted types of vegetables in all your meals. Vegetables are essential and should not just be a side dish but the centerpiece of your meal.
- Consuming foods rich in fiber like grains, beans, peas, nuts, and seeds.
- Consuming less red meat, like pork, lamb, goat, and bison.
- Including probiotic and prebiotic foods to support a healthy gut. Probiotic foods include yogurt or other fermented vegetables, and prebiotic foods include raw or cooked onions, dandelion greens, and legumes.
- Avoiding processed and canned foods.
- Avoiding foods high in calories and low in nutrients like fruit-flavored drinks, sodas, candies, and sweets.
- Cutting down on the excessive intake of alcohol.
Exercise and Cancer Care
Other routines that can help improve cancer care include regular exercise. Regular exercise helps to control fatigue, muscle tension, and anxiety in those with cancer, and it is essential, especially during and after cancer treatment, to keep fit and avoid gaining unnecessary weight.
Cancer Treatment Options
There are many cancer treatment options for different types of cancers. Cancer treatments depend on the type and how advanced it is. Some patients with cancer will only have one treatment option, while others will end up with a combination of more than one treatment technique depending on the type and stage of cancer.
Curing cancer is one of the significant challenges of the 21st century. However, few advances to tame the immune system are getting closer to a future where cancer can be a curable disease.
Advances in Immunotherapy
Although cancer treatment is dependent on the stage and type, we need a wide range of therapies that work on a cellular level, like NK cell and stem cell therapies, wide enough to cover the whole spectrum of cancer. Cancer therapies involving the immune system are believed to be a milestone for cancer treatment advances and the development of multiple immune cells as a therapeutic tool.
Our knowledge of cancer has dramatically improved in recent years. It seems increasingly evident that there has been a surge of new technologies, and these technologies could make a great impact on the way we treat cancer, taking us closer to finally curing this deadly disease.
NK Cells: A Promising Cancer Treatment
What Are NK Cells?
One of these promising cancer treatment advances is the natural killer (NK) cell-based immunotherapy. Natural killer cells have emerged as the newest promising therapeutic approach to cancer. Our understanding of the biological processes that take place in cancer is increasing rapidly, leading to this new type of targeted treatment and therapeutic approach. It is hard to overstate the possible importance of NK cells in the future of cancer.
How NK Cells Work
Modern research has shown that NK cells are a type of treatment that stimulates a person’s immune system to fight cancer, and this may give room for a greater number of chances to beat cancer for good. NK cells are also known for their ability to target cancer stem cells, making these cancer stem cells visible to the immune system.
NK cells were first identified in the 1970s as a unique lymphocyte subset that can recognize and kill abnormal cells without prior sensitization to specific tumor antigens, thus preventing the growth of many cancers. During the late 1980s, it was observed that NK cells could destroy a lymphoma cell line that had lost MHC class I surface molecules while the original MHC class I+ cells were resistant to lysis. This led to the formation of the “missing self-hypothesis,” which states that NK cells can sense the absence of “self” MHC class-I molecules on target cells. In recent years, the hypothesis was confirmed by the inhibitory and activating NK cell receptors.
Mechanisms of NK Cell Action
This discovery indicates that autologous cells are not killed by NK cells, thanks to an appropriate expression of all self-HLA alleles, preventing the killing of autologous cells. In contrast, a wide spectrum of cancer types can be killed due to the loss of HLA molecules and the expression/overexpression of ligands for NK cell activating receptors.
NK cells first recognize the tumor cells via stress or danger signals from their sensors. Then, the activated NK cells directly decimate the target tumor cells through at least four mechanisms: cytoplasmic granule release, death receptor-induced apoptosis, effector molecule production, or antibody-dependent cellular cytotoxicity (ADCC).
Furthermore, NK cells act as regulatory cells when they reciprocally interact with dendritic cells (DCs) to improve their antigen uptake and presentation. This action helps in facilitating the generation of antigen-specific cytotoxic T lymphocyte (CTL) responses that are vital in dictating foreign invasion and tumor cells.
By producing cytokines such as IFN-γ, activated NK cells induce CD8+ T cells to become CTLs. Activated NK cells can also help in the differentiation of CD4+ T cells toward a Th1 response and promote CTL differentiation. Cytokines produced by NK cells might even have the unique ability to regulate antitumor cells, making them more proactive.
Enhancing NK Cell Therapy
Accordingly, the cytokine gene transfer approach induces NK cell proliferation, increasing survival capacity, further enhancing their activation, and making them more potent in malignant cell multiplication dictation. NK cell scientists have assured that by using NK cell lines and modifying genes such as IL-2, IL-12, IL-15, and stem cell factor (SCF), they have demonstrated the ability of NK cells to restore their cytotoxic capacity as well as increase their proliferative rate, survival ability, and in vivo antitumor activity. However, the specificity of NK cells is still limited, and studies are still passing through phases. The approach focuses on retargeting NK cells to tumor cells by gene transfer of chimeric tumor-antigen specific receptors.
NK therapy is promising research; it raises the prospect of a “one-end-solution” to many different types of cancers across the globe. If the studies and experiments work out well, NK cells will be able to increase the activity of CD4+, CD19, and other important disease-fighting cells of the body.
The Future of NK Cell Therapy
There is no doubt that this is an unimaginable feat, both in advancing our basic knowledge about regenerative medicine and for the possibility of future cancer treatment advances.
- Published in Corporate News / Blog
Clinical trials on NK cells give hope for many people who are suffering from cancer.
Induced Pluripotent Stem Cells (iPSCs)
Induced pluripotent stem cells (also known as iPS cells or iPSCs) are a type of pluripotent stem cell that can be generated directly from a somatic cell. Pluripotent stem cells hold promise in the field of regenerative medicine. Because they can propagate indefinitely, as well as give rise to every other cell type in the body (such as neurons, heart, pancreatic, and liver cells), they represent a single source of cells that could be used to replace those lost to damage or disease.
Natural Killer Cells and Their Role
Natural killer (NK) cells are a type of cytotoxic lymphocyte critical to the innate immune system. The role NK cells play is analogous to that of cytotoxic T cells in the vertebrate adaptive immune response. NK cells provide rapid responses to virus-infected cells, acting at around three days after infection, and respond to tumor formation.
Typically, immune cells detect the major histocompatibility complex (MHC) presented on infected cell surfaces, triggering cytokine release and causing apoptosis. NK cells are unique, however, as they can recognize stressed cells in the absence of antibodies and MHC, allowing for a much faster immune reaction.
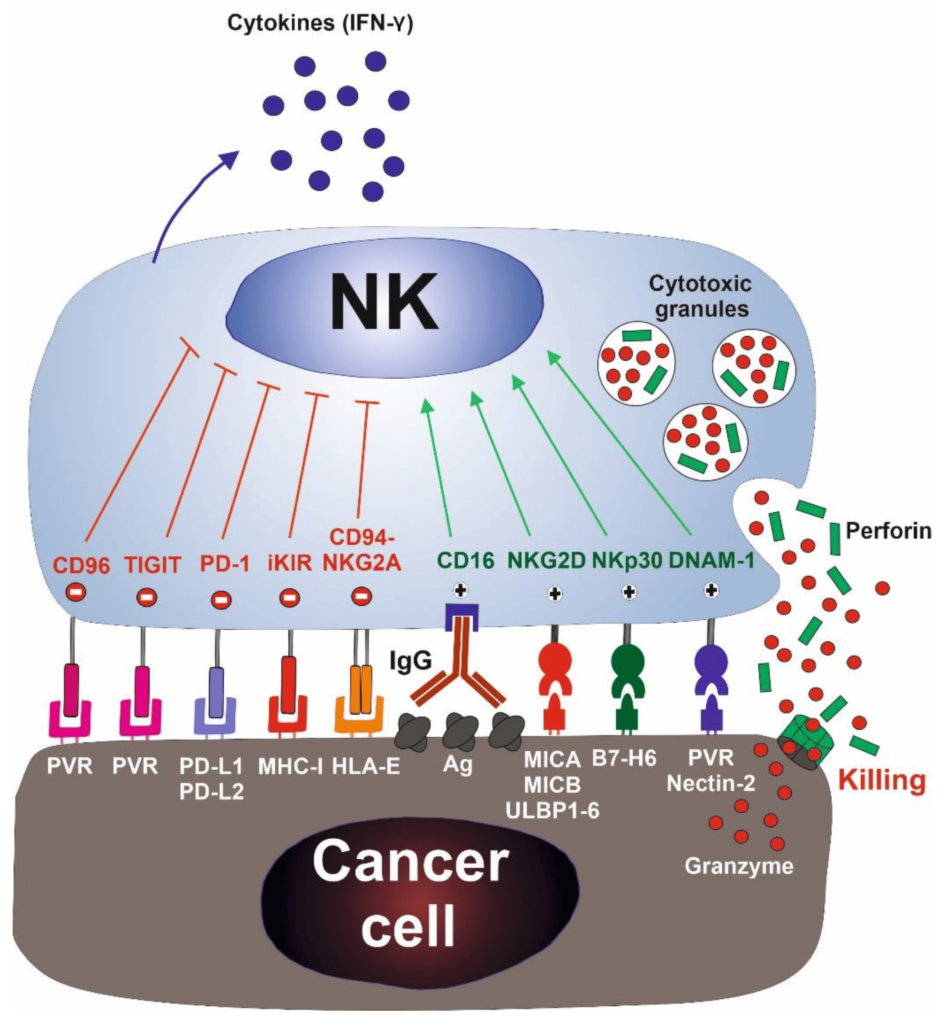
Clinical Trials on NK Cells
Overview of NK Cell Clinical Trials
In a first clinical trial, a natural killer cell immunotherapy derived from induced pluripotent stem cells is being tested for safety in 64 patients with a variety of solid tumors. The first subjects used for the study received the cells in February at the University of California, San Diego (UCSD) Moores Cancer Center and MD Anderson Cancer Center.
Targeted Cancer Types
This study targets late-stage cancer patients with solid tumors, including lymphoma, colorectal cancer, and breast cancer. The FT500 NK cells do not undergo any further alterations after their derivation from induced pluripotent stem cells (iPSCs), offering the possibility of a quicker, ready-made treatment.
Human Embryonic Stem Cells and iPSCs
Human embryonic stem cells (hESCs) and induced pluripotent stem cells (iPSCs) provide an accessible, genetically tractable, and homogenous starting cell population to efficiently study human blood cell development. These cell populations provide platforms to develop new cell-based therapies to treat both malignant and nonmalignant hematological diseases.
NK Cells and Cancer Cell Targeting
The NK cells are immune cells in the same family as T and B cells and are very good at targeting cancer cells for destruction. Laboratory experiments have shown they do so by attacking cells that have lost their significant self-recognition signals that tell the immune system not to attack. This is the phenomenon that can happen among cancer cells but not healthy cells. Experts are not sure how many cancer cells lose that signal. Researchers are hopeful that the clinical trial can help determine which cancer patients could benefit the most from NK cell treatment.
iPS Clones and Tumor Formation
The ability to induce pluripotent stem cells from committed, human somatic cells provides tremendous potential for regenerative medicine. However, there is a defined neoplastic potential inherent to such reprogramming that must be understood and may offer a model for critical understanding of events in the formation of tumors. Using genome-wide assays, we identify cancer-related epigenetic abnormalities that arise early during reprogramming and persist in induced pluripotent stem cell (iPS) clones. These include hundreds of abnormal gene silencing events, patterns of aberrant responses to epigenetic-modifying drugs resembling those for cancer cells, and the presence in iPS and partially reprogrammed cells of cancer-specific gene promoter DNA methylation alterations.
Modification of T Cells for Cancer Therapy
Progress in Adoptive T-cell Therapy
Progress in adoptive T-cell therapy for cancer and infectious diseases is hampered by the lack of readily available antigen-specific human T lymphocytes. Pluripotent stem cells could provide an estimable source of T lymphocytes, but the therapeutic potential of human pluripotent stem cell-derived lymphoid cells generated to date remains uncertain.
CAR T-Cell Therapy
Recently, some approved cell therapies for cancer also rely on modifying T cells, in those cases to produce cancer cell–binding chimeric antigen receptors (CARs), and have been effective in treating certain cancers such as leukemia.
Application of CAR T-Cell Therapy in Solid Tumors
The CAR T technology has wowed the field by all but obliterating some patients’ blood cancers, but solid malignancies present new challenges.
Therapies containing such chimeric antigen receptor (CAR) T cells have been approved for some types of so-called liquid cancers of the blood and bone marrow, such as large B-cell lymphoma and B-cell acute lymphoblastic leukemia. However, the approach has not had as much success for solid tumors.
Challenges and Innovations
Serious research into the therapy for brain cancer started almost 20 years ago after cancer biologist Waldemar Debinski, then at Penn State, discovered that the receptor for the immune signaling molecule interleukin 13 (IL-13) was present on glioblastomas, but not on healthy brain tissue. The receptor thus seemed like an excellent target to home in on cancer cells while sparing healthy ones. The CAR spacer domain that spans the immune cells’ membranes and its intracellular co-stimulatory areas, as well as the process used to expand cells outside the body, boosts the T cells’ activity.
CAR T-Cell Therapy: A Safer Approach
While managing CAR T-cell therapy toxicity could help keep already-designed treatments on their march to the clinic, many immunotherapy companies are also working to develop a new generation of inherently safer therapies, yet just as efficient. A crucial part of achieving this goal will be improving CAR specificity for target cells. With current treatments, the destruction of normal cells is often an unavoidable side effect when healthy tissue carries the same antigens as tumors; noncancerous B cells, for example, are usually casualties in CD19-targeted therapies.
Delivery Challenges
CAR T delivery is a challenging factor in the treatment of solid tumors and other unknown forms of tumors. With non-solid cancers, cells are administered by a blood infusion, and once in circulation, the CAR T cells can seek out and destroy the rogue cells. For solid tumors, it’s not so simple.
Future Directions
The main drawback of taking cells from a patient and developing them into a cellular immunotherapy product is that the process can take weeks.
Patel tells The Scientist, “But for the majority of patients who may not be a candidate or may not have time to wait for such an approach, the idea that there’s off-the-shelf immunotherapy that could potentially act as a living drug against their cancer, I think is a fascinating concept.”
- Published in Corporate News / Blog
Stem cell treatment could offer one-end-solution to Diabetes
Insulin-producing cells grown in the lab could provide a possible cure for the age-old disease, diabetes.
Understanding Type 1 Diabetes
Type 1 diabetes is an autoimmune disease that destroys insulin-producing pancreatic beta cells, leading to dangerously high blood glucose levels. Patients often require insulin administration and other medications to manage blood sugar levels. For those who struggle with blood sugar control, beta-cell transplants are an option, but they require immunosuppressive drugs.
Breakthroughs in Stem Cell Research
A research group at Harvard University reported using insulin-producing cells derived from human embryonic stem cells (ESCs) and induced pluripotent stem cells to lower blood glucose levels in mice. Laboratories worldwide are making rapid progress in human stem cell technology to develop cells functionally equivalent to beta cells and other pancreatic cell types. Novel biomaterials are being developed to encapsulate these cells, protecting them from the immune system without the need for immunosuppressants.
Major Investments in Diabetes Treatment
Pharmaceutical companies and venture capital firms have invested over $100 million in leading biotechnology companies to bring stem cell treatments into clinical use. Prominent companies include:
- Semma Therapeutics
- Sigilon Therapeutics
- ViaCyte
Transforming Human Stem Cells into Insulin-Producing Cells
Researchers at UC San Francisco have successfully transformed human stem cells into mature insulin-producing cells, a significant breakthrough in developing a cure for Type 1 Diabetes (T1D). These cells mimic the function of pancreatic beta cells and offer hope for creating transplantable cells for patients with diabetes.
What is T1 Diabetes?
Type 1 diabetes is characterized by the destruction of insulin-producing beta cells in the pancreas, typically starting in childhood. Without insulin, blood glucose levels can spike, causing severe organ damage and potentially leading to death. The condition can be managed with insulin shots, but serious health complications often persist, such as kidney failure, heart disease, and stroke.
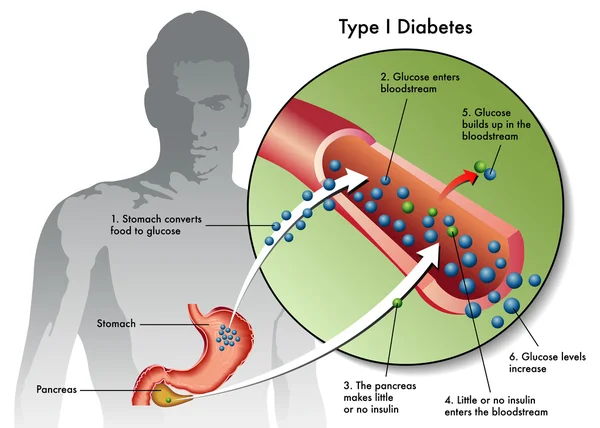
Making Insulin-Producing Cells from Stem Cells
Diabetes can be treated through a pancreas transplant or donor cell transplantation, but both methods rely on deceased donors. Scientists have struggled to produce mature beta cells responsive to blood glucose and capable of secreting insulin correctly. However, recent breakthroughs at UCSF have achieved this transformation in mice, marking a critical step toward human application.
Protecting Stem Cell Therapies from the Immune System
Delivering stem cell therapies requires protection from immune attacks while maintaining cell functionality. Companies are exploring two main strategies:
- Microencapsulation: Immobilizing cells individually or in small clusters within biocompatible gel blobs.
- Macroencapsulation: Placing larger numbers of cells into a larger, implantable device.
Companies Leading the Way
- ViaCyte: Partnered with Johnson & Johnson, using microencapsulation in clinical trials.
- Semma: Developing both macro- and microencapsulation methods, readying for clinical trials.
- Sigilon: Utilizing gel-based spheres for encapsulation, in collaboration with Eli Lilly.
Conclusion
Stem cell treatment holds the potential to revolutionize diabetes care, offering a one-end solution to managing and potentially curing the disease. With significant investments and ongoing research, the future of diabetes treatment looks promising. Researchers and companies are making strides in developing effective and safe stem cell therapies, bringing hope to millions worldwide.
- Published in Corporate News / Blog
Bats Carry Corona Virus. So Why Don’t They Get Sick?
A lot of viruses that have taken a toll on life, such as the Ebola virus in Africa, the Nipah virus, and the most recent coronavirus that left China running helter-skelter, all seemed to have originated from bats.
Origins of the Coronavirus
During the course of the virus epidemic in Wuhan, where it was first detected, some Chinese researchers in Wuhan examined some patients affected in that area and took samples of the virus. They did findings on the genetic sequence of the virus with other viruses that were known. The coronavirus surprisingly had a 96% match with the horseshoe bats that are dominant in the southwest of China. The research findings were then published in a study in February 2020.
A virologist Vineet Menachery from the University of Texas Medical Branch at Galveston, though not affiliated with the study, said, “They’re too close in terms of their pure genetics to say they’re not related, or that they didn’t have a common ancestor.” Menachery was a reputable virologist and had done other research works. He contributed to the theory that the spread of the coronavirus must have been from these bats to humans and possibly must have had another animal that served as an intermediary for the spread.
Similar Cases of Virus Transmission
This same thing had happened with other forms of coronaviruses as noted in the case of SARS (Severe Acute Respiratory Syndrome), an outbreak that took place in 2002-2003 where civets, a mongoose family member, were infected with the bat coronavirus and spread as humans bought them for food. Another case was the MERS (Middle East Respiratory Syndrome) outbreak. This one happened in 2012 and was a result of infected camels from the virus. People who ate undercooked meat of camels and drank the raw milk of camels were all affected.
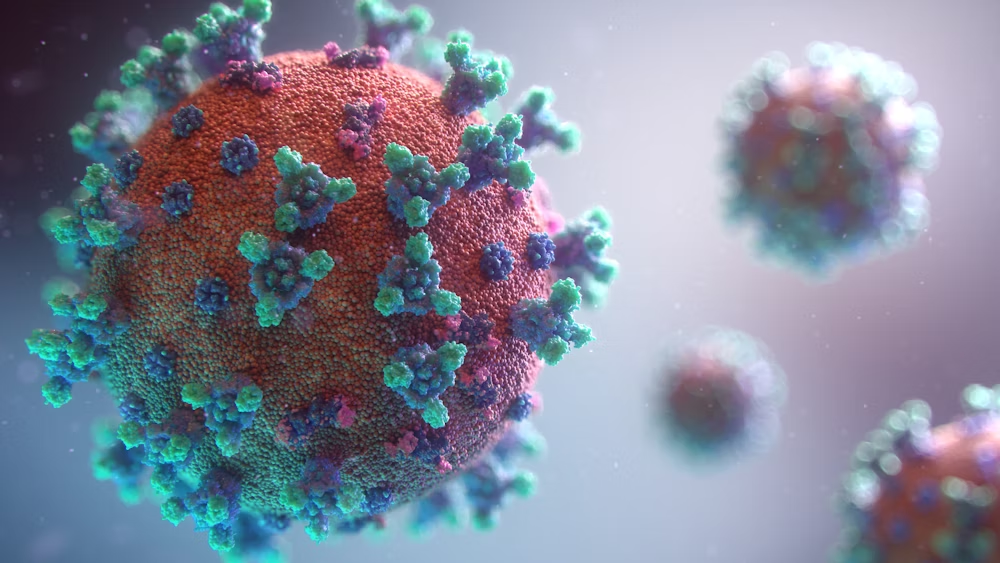
Why Don’t Bats Get Sick?
So why is it that there are so many diseases that are spread from bats? It’s no doubt, bats have a lot of viruses that they carry with them. And these viruses, in their variety, are spread and manifest their tolls on people. Scientists are not sure why this is the case as confirmed by Kevin Olival, a research vice president at EcoHealth Alliance, a non-profit organization based in the U.S. He went further to say that it may have something to do with the family of the viruses carried by the bats. There are over 130 different families of viruses that bats do carry around. And then, most bats and humans do come in contact through several means. The millions of populations of bats are ubiquitous to all the continents apart from Antarctica. Rebekah of Colorado State University who researched infectious pathogens said, “There’s a lot of viruses we’re finding in bats because there’s a lot of bats out there.”
Bats and Their Unique Biology
Bats move about in multitudes and live in colonies of large populations. Some of these members live in caves and share caves and trees where there can be contact between humans and bats. Hence, these viruses can spread from these bats to humans. Despite their sizes, bats have relatively long lifespans and can live over 30 years. “So there’s a long time for them to be persistently infected with the virus and shed it into the environment,” Kading says. The modes of mechanisms for these viruses are through urine, saliva, and feces of bats. The outbreak of Nipah that happened in Bangladesh was linked to the sap of a date palm gotten from some trees that some bats licked and had infested with their urine.
Reading through all these, it is not absurd to wonder why the bats themselves do not get affected by the viruses they carry. The answer to that question is based on the fact that the bat is the only flying mammal in the world. Their body metabolism and processes quite differ from those of normal mammals too. When bats fly, their heart rates rise to about a thousand beats per minute with a temperature rise of about 100 degrees Fahrenheit. Linfa Wang, a student of bat viruses at Duke-NUS Medical School in Singapore, says that when these signs manifest in other mammals, they are signals that can trigger death. But this is not the same case for bats. This is a lifestyle for them every day. Their system is also capable of producing molecules that other organisms do not have. The molecules carry out repair functions and prevent cell damage. This makes their system a bit irresistible to infections and also makes them recalcitrant to viruses and resilient to diseases such as diabetes, cancer, and other health conditions. This is proof that the manifestation of viruses in mammals is not always as a result of the virus itself but as a result of the body’s reaction to the presence of such a virus that makes us ill by triggering other chain reactions, as Wang explains.
Human Activities and Virus Transmission
Olival at EcoHealth explains that these bats have coevolved with these viruses and it is not totally their fault that we humans are infected and affected by these viruses. The actual problem is when the viruses move from their species to other species of mammals which is also fostered by human activity. Naturally, it would be hard for most animals and mammals to cross paths. But Olival says that the presence of some activities and availability of exchange platforms made available by humans can allow such interaction to occur. She gave an example using wildlife markets like the one in Wuhan, where a bat could be mixed up with a civet, which later on comes in contact with humans – e.g., butchers who do not observe proper hygiene and protection from animal blood.
“The way that we’re coming into contact with these animals, hunting, selling, and trading them is to a scale that really we haven’t seen before,” he says. Investigative teams did some in-depth search and they discovered some traces of this virus in 22 stalls and in a garbage truck that was found at Huanan Seafood Market right there in Wuhan, a place known for booming trade for live animals. This discovery led to shutting down the market as it was tied to the majority of the cases.
Conclusion
The intermediary animals to these viruses are still a mystery, but it is clear that some of these animals are prone to interact more with humans. This is why when they are infected, the likelihood for human infection is widened. These other infected animals can sneeze, urinate, be cooked as food, or even owned as pets. Bats are not just vectors for viruses, they play an important role in balancing the ecosystem. They feed on insects and fruits and are active agents of pollination. In fact, Wang believes that since these bats have successfully coevolved with these viruses, there is every possibility that they can be the agents that can lead to the cure and provision of therapies for these viruses.
- Published in Corporate News / Blog
A New Medical Device, in the Management of Complex Wounds
Innovative Wound Healing: The Gcells Solution
Because of the complex nature of the wound healing process, an injury on the skin can pose several challenges and likely complications, especially when acute. They can deteriorate from acute to chronic conditions, requiring external intervention best understood by a specialist physician to restore normalcy to the affected area.
The Complexity of Wound Healing
The complexity of wound healing and research remains an ocean of knowledge that is continuously researched intensely to uncover depths of wound healing techniques and interventions. Hence, this report contains an introduction and details on the use of a new medical innovation called Gcells, used primarily for the management of wounds of different etiologies.
Gcells: A Breakthrough in Wound Management
In cases where the process of wound healing seemed difficult, Gcells proved great effects, attributed to their design and working protocol. Gcells are conditioned to work with an enriched suspension of progenitor cells that can efficiently aid the tissue repair process. In this case report, two subjects were used as donors and acceptors of these micro-grafts.
Understanding the Skin’s Repair Mechanism
The skin is the outer layer of the body, offering protection to the underlying layers. A wound breaks this layer, inhibiting its various functions and potentially exposing or breaking the underlying tissue layers. Repair processes are inherent and part of homeostatic processes of the body to try to restore the skin to its normal structure and function.
Basic Wound Healing Process
The basic process for skin repair involves clot formation and inflammation, where vessels dilate and monocytes activate, leading to the breakdown of necrotic tissues. This basic process can be inhibited or delayed by various factors, leading to the transformation of acute wounds to chronic forms. If there is no alteration in the repair process, mesenchymal cells kickstart the proliferative process, beginning to repair and restructure the affected tissues from the base. At the same time, epithelial tissues begin to grow around the wound, leading to the final step of the healing process: skin remodeling and scar maturation.
Factors Affecting Wound Healing
These processes work best under certain conditions. Factors such as cardiovascular ailments, diabetes, bacterial infections, or other types of infections can inhibit these processes.
Importance of Mesenchymal Cells
During the proliferative phase of wound healing, mesenchymal cells are key players. Their structure includes mesenchymal stem cells (MSCs), which are multipotent and offer supportive, therapeutic, and trophic functions. They can release viable trophic, anti-inflammatory cytokines, and anti-apoptotic molecules that offer protection during the repair of wounded skin. MSCs also possess subpopulations with stem-like nature, commonly referred to as “side population” (SP) cells. These cells are enriched in progenitor and multipotent stem cells and exist in various tissues and tumors.
Research on Stem Cells
Research has shown that these stem cells can differentiate into osteoblasts and build a woven bone by forming an extracellular matrix (ECM) secreted by the osteoblasts. This discovery demonstrated the potential of dental pulp as a rich source of progenitor/autologous cells that can aid in healing processes, including the regeneration of craniofacial bones.
Gcells Innovation: Working Principle
Gcells successfully separate this side population with a size of 50 microns. At this cell population, they can form autologous micro-grafts that can be used alone or alongside biomaterials prepared in a biocomplex ready for use when necessary.
Case Report Overview
In this case report, two subjects were used as donors and acceptors of these micro-grafts for enhanced healing of complex wounds through autologous micro-grafts using Gcells.
Clinical Case 1: Abdominoplasty Bariatric Complications
The first case involves a 50-year-old woman with no diseases or disorders. She underwent a laparoscopic gastric bypass surgery and was doing well with weight loss parameters. Two years later, she moved in for abdominoplasty bariatric surgery. Post-surgery complications showed necrosis, discovered after the first medical examination, with tissue loss of about 150 to 200cm² at the end of the flaps.
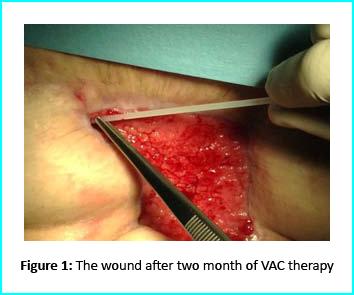
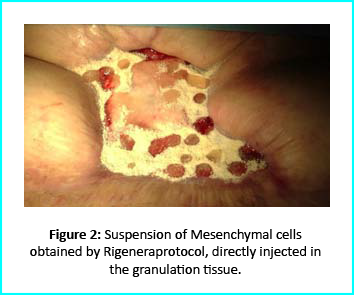
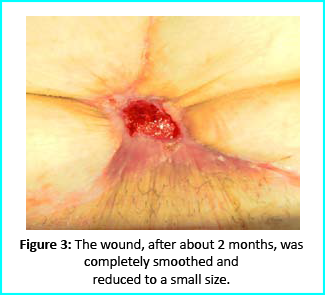
Initial Treatment
An initial necrosectomy showed an intense loss of tissue, and the wound was placed on VAC therapy. The patient actively participated in this therapy for one week, then continued at home as an outpatient. The VAC therapy showed progressive wound cleansing and granulation tissue formation around the base area.
Gcells Intervention
The Gcells protocol started after consent from the outpatient. A 3cm² skin sample was collected from the patient to obtain the cell suspension needed to be injected into the granulation tissue. Conventional wound treatment followed, including cleaning and replacement with sterile gauze dabbed with Vaseline.
Outcome
The wound area began to improve in healing progress and general appearance. In two months, the undermined area disappeared and leveled with the skin surface. Two months later, the wound reduced to a very mild and smooth scar compared to the initial condition.
Clinical Case 2: Complications from Liver Cirrhosis and Surgery
The second case involves a 78-year-old man who suffered from liver cirrhosis, hiatal hernia, and diabetes. He underwent complex surgery, including distal esophagectomy and ileostomy protection. Post-surgery complications included necrotic tissues around the biological prosthesis.
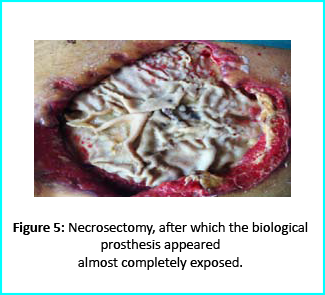
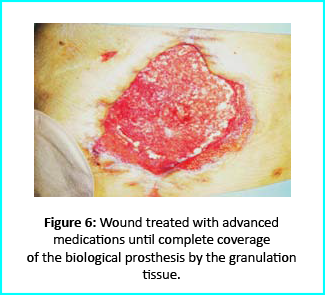
Initial Treatment
Necrosectomy was conducted, leaving the biological prosthesis half exposed. Further treatment helped cover the biological prosthesis with granulation tissue. Plastic surgeons evaluated the patient, and VAC therapy was used on the wound for about 15 days.
Gcells Intervention
The Gcells protocol was applied when the wound dimension progressed to about 250cm². The tissue granulation near the ileostomy improved, though it appeared undermined.
Outcome
Gcells protocol proved highly effective in healing and restoring damaged tissues. This progress opens the way for its use in clinical practice for treating and managing acute and chronic wounds and other medical fields needing tissue repair.
Discussion and Conclusion
Gcells protocol has demonstrated efficiency in aiding wound healing, especially for wounds likely to develop from acute to chronic conditions. The working principle of Gcells, using one individual as both the donor and acceptor, reduces complications related to non-autologous implants or micro-grafts. Gcells are flexible and can be used in operating rooms and ambulatories. This innovation is spreading and is currently used in oral-maxillofacial fields, plastic surgery, dermatology, and orthopedics.
Final Thoughts
Gcells offer a promising, efficient, and low-risk innovation in medicine for wound management and healing. The device still needs further testing on subjects with different conditions, but its potential to improve healing of complex wounds is significant. Gcells can reduce the use of expensive devices and offer employment in clinical procedures aiding in wound management.
- Published in Corporate News / Blog
Conventional and novel stem cell based therapies for androgenic alopecia
Dodanim Talavera-Adame,1 Daniella Newman,2 Nathan Newman1
1 American Advanced Medical Corp. (Private Practice), Beverly Hills, CA
2 Western University of Health Sciences, Pomona, CA, USA
Abstract
The prevalence of androgenic alopecia (AGA) increases with age and it affects both men and women. Patients diagnosed with AGA may experience decreased quality of life, depression, and self-consciousness. There are various therapeutic options ranging from prescription drugs to non-prescription medications. Currently, AGA involves an annual global market revenue of US$4 billion and a growth rate of 1.8%, indicating a growing consumer market. Although natural and synthetic ingredients can promote hair growth and, therefore, be useful to treat AGA, some have significant adverse effects and unknown mechanisms of action that limit their use and benefits. Biological factors including signaling from stem cells, dermal papilla cells, and platelet-rich plasma are some of the current therapeutic agents being studied for hair restoration with milder side effects. However, most of the mechanisms exerted by these factors in hair restoration are still being researched. In this review, we analyze the therapeutic agents that have been used for AGA and emphasize the potential of new therapies based on advances in stem cell technologies and regenerative medicine.
Introduction
The prevalence of androgenic alopecia (AGA) increases with age and is estimated to affect about 80% of Caucasian men.1 Female AGA, also known as female pattern hair loss, affects 32% of women in the ninth decade of life.2 The consumer market for products that promote hair growth has been increasing dramatically.3 These products promote hair regeneration based on the knowledge about the hair follicle (HF) cycle.4,5 However, in most cases, the mechanisms of action of these products are not well characterized and the results are variable or have undesirable side effects.6 At present, only two treatments for AGA have been approved by the US Food and Drug Administration (FDA): Minoxidil and Finasteride.7–10 Although these medications have proved effective in some cases, their use is limited by their side effects.11,12 With the emergence of stem cells (SCs), many mechanisms that lead to tissue regeneration have been discovered.13 Hair regeneration has become one of the targets for SC technologies to restore the hair in AGA.14 Several SC factors such as peptides exert essential signals to promote hair regrowth.15,16 Some of these signals stimulate differentiation of SCs to keratinocytes, which are important for HF growth.17 Other signals can stimulate dermal papilla cells (DPCs) that promote SC proliferation in the HF.18,19 In this review, we describe HF characteristics and discuss different therapies used currently for AGA and possible novel agents for hair regeneration. These therapies include FDA-approved medications, non-prescription physical or chemical agents, natural ingredients, small molecules, biologic factors, and signals derived from SCs.
Hair Follicle and Stem Cell Niche
The HF undergoes biologic changes from an actively growing stage (anagen) to a quiescent stage (telogen) with an intermediate remodeling stage (catagen).4 HFSCs are located in the bulge region of the follicle and interact with mesenchymal SCs (MSCs) located in the dermal papilla (DP).18 These signal exchanges promote activation of some cellular pathways that are essential for DPC growth, function, and survival, such as the activation of Wnt signaling pathway.19–21 Other signals, such as those from endothelial cells (ECs) located at the DP, are also essential for HF maintenance.22 EC dysfunction that impairs adequate blood supply may limit or inhibit hair growth.22 For instance, Minoxidil, a synthetic agent, is able to promote hair growth by increasing blood flow and the production of prostaglandin E2 (PGE2).7 It has been shown that proteins belonging to the transforming growth factor (TGF) superfamily, such as bone morphogenetic proteins (BMPs), also exert signals to maintain the capacity of DPCs to induce HF growth in vivo and in vitro.23 These BMPs may be released by several cells composing the follicle, including ECs.24–26 ECs may provide signals for BMP receptor activation in DPCs similar to those signals that promote survival of MSCs in human embryoid bodies composed of multipotent cells.24,25 DPCs have been derived from pluripotent SCs to study their potential for hair regeneration in vitro and in vivo.27 Together, dermal blood vessels and DPCs orchestrate a suitable microenvironment for the growth and survival of HFSCs.28,29 Interestingly, the expression of Forkhead box C1 regulates the quiescence of HFSCs located in the bulge region.30 HFSCs are quiescent during mid-anagen and maintain this stage until the next hair cycle.29,30 However, during the early anagen stage, these cells undergo a short proliferative phase in which they self-renew and produce new hair.30 Therefore, the bulge region constitutes an SC niche that makes multiple signals toward quiescence or proliferation stages.30–34 It is known that fibroblasts and adipocyte signals can inhibit the proliferation of HFSCs.34 Additionally, BMP6 and fibroblast growth factor 18 (FGF18) from bulge cells exert inhibitory effects on HFSC proliferation.34 Dihydrotestosterone (DHT) also inhibits HF growth.35 Agents that reduce DHT, such as Finasteride, promote hair regrowth by inhibiting Type II 5a-reductase.8,14,36 In contrast to these inhibitory effects, DPCs located at the base of the HF provide activation signals.18,34 The crosstalk between DPCs and HFSCs leads to inhibition of inhibitory effects with the resultant cell proliferation toward hair regeneration (anagen).30,31,37 With the self-renewal of HFSCs, the outer root sheath (ORS) forms, and signals from DPCs to the bulge cells diminish in a way that the bulge cells start again with their quiescent stage.4,34 As mentioned earlier, the Forkhead box C1 transcription factor has an important role in maintaining the threshold for HFSC activation.30 The knockdown of these factors in bulge cells reduces the cells’ threshold for proliferation, and the anagen cycle starts more frequently due to promotion of HFSC proliferation in shorter periods of time.30
Prescribed and Non-Prescription Products that Promote Hair Growth
FDA-Approved Chemical Agents
At present, the only therapeutic agents for AGA approved by the FDA in the USA are Finasteride and Minoxidil.9,10 Minoxidil promotes hair growth by increasing the blood flow and by PGE2 production.7 Although Minoxidil is now a non-prescription medication, Finasteride and other drugs require a medical prescription for AGA treatment (Table 1). Dutasteride and Finasteride inhibit 5a-reductase, blocking the conversion of testosterone to DHT.36,38 While Finasteride is a selective inhibitor of type II 5a-reductase, Dutasteride inhibits type I and type II 5a-reductases. These medications have also been used to treat benign prostatic hyperplasia.
Table 1: Prescribed Products Used for AGA
Prescribed Products | Source | Mechanism of Action |
---|---|---|
Finasteride/Dutasteride | Synthetic (small molecule) | Inhibits type II, 5a-reductase |
Latanoprost and Bimatoprost | Synthetic prostaglandin analog of PGF2a | Activates prostaglandin receptor |
Natural Ingredients for Hair Growth
In addition to prescribed medications, some natural ingredients have been used to promote hair growth. These ingredients have various mechanisms of action that can help in managing androgenic alopecia (AGA).
Procyanidin B-2
Procyanidin B-2, found in apples and several plants, can inhibit the translocation of protein kinase C (PKC) in hair epithelial cells. PKC isozymes, such as PKC-ßI and -ßII, play an important role in hair cycle progression. Inhibiting their translocation can promote hair growth.
Procyanidin B-3
Procyanidin B-3 promotes hair growth by inhibiting TGF-ß1.
Other Natural Ingredients
Other natural ingredients such as saw palmetto, alfatradiol, and green tea (Epigallocatechin gallate) have the capacity to inhibit 5a-reductase and block DHT production.
Summary of Natural Ingredients
The natural ingredients and their proposed mechanisms of action are summarized in Table 2. The commercial web page is included since there are no formal studies about their mechanisms of action.
Abbreviations
AGA: Androgenic alopecia
PKC: Protein kinase C
TGF-ß1: Transforming growth factor beta 1
DHT: Dihydrotestosterone
Table 2: Natural Ingredients Used for Hair Growth
Natural Ingredient | Mechanism of Action |
---|---|
Procyanidin B-2 | Inhibits the translocation of protein kinase C (PKC) in hair epithelial cells |
Procyanidin B-3 | Inhibits TGF-ß1 |
Saw palmetto | Inhibits 5a-reductase and blocks DHT production |
Alfatradiol | Inhibits 5a-reductase and blocks DHT production |
Green tea (Epigallocatechin gallate) | Inhibits 5a-reductase and blocks DHT production |
Table 2: Non-Prescription Products Used for AGA and Their Proposed Mechanisms of Action
Non-Prescription Product | Source | Proposed Mechanism of Action |
---|---|---|
Minoxidyl (FDA approved) | Synthetic (small molecule) | Potassium channel opener and powerful vasodilator used in hypertension |
Apple Procyanidin B-2 | Natural (apples and plants) | Inhibitor of translocation of PKC isozymes in hair epithelial cells |
Procerin | Natural (saw palmetto extract) | Inhibits type I and II 5a-reductase and blocks DHT production |
Provillus | Formulation | Contains Minoxidil and vitamins, similar to Procerin |
Follicusan | Natural (milk-based compound) | Stimulates cellular functioning in the scalp and hair follicle; improves hair density and thickness |
Musol 20 | Natural (yeast extract) | Physically deposited as a protective covering to create thicker hair |
Capixyl | Synthetic and natural | Inhibitor of 5a-reductase; improves ECM proteins and reduces inflammation |
EMortal Pep | Synthetic and natural | Blocks upregulation of TGF-ß1 induced by DHT; activates dermal papilla cells |
Planoxia-RG | Natural | Promotes transition from telogen phase to anagen phase |
Tricholastyl | Natural (plant extracts) | Antiglycation activity; restores the hair growth cycle |
Keramino-25 | Synthetic | Increases the strength of the hair due to enhanced penetration |
Seveov | Natural (maca root extract) | Protects the hair bulb and shaft; stimulates cell division in the hair shaft and bulb |
Hairomega | Natural (saw palmetto and ß-sitosterol) | Inhibits 5a-reductase and formation of DHT |
Green tea (Epigallocatechin gallate) | Natural (polyphenol antioxidant) | Inhibits 5a-reductase and formation of DHT |
Nioxin | Synthetic (Coenzyme Q10 and coenzymes) | Inhibits 5a-reductase and formation of DHT |
Alfatradiol | Synthetic (17a-estradiol) | Inhibits type II 5a-reductase |
Quercetin | Natural (flavonoid) | Inhibits PGD2 |
Laser Therapy
Light amplification by stimulated emission of radiation (LASER) generates electromagnetic radiation which is uniform in polarization, phase, and wavelength. Low-level laser therapy (LLLT), also called “cold laser” therapy, utilizes lower power densities than those needed to produce heating of tissue. Transdermal LLLT has been used for therapeutic purposes via photobiomodulation. Several clinical conditions, such as rheumatoid arthritis, mucositis, pain, and other inflammatory diseases, have been treated with these laser devices. LLLT promotes cell proliferation by stimulating cellular production of adenosine triphosphate and creating a shift in overall cell redox potential toward greater intracellular oxidation. The redox state of the cell regulates activation of signaling pathways that ultimately promote high transcription factor activity and gene expression of factors associated with the cell cycle.
Physical agents such as lasers have also been used to prevent hair loss in a wavelength range in the red and near infrared (600–1,070 nm). Laser therapy emits light that penetrates the scalp and promotes hair growth by increasing blood flow. This increase gives rise to endothelial cell proliferation and migration due to upregulation of vascular endothelial growth factor (VEGF) and endothelial nitric oxide synthase. In addition, the laser energy itself stimulates metabolism in catagen or telogen follicles, resulting in the production of anagen hair. A specific effect of LLLT has been demonstrated to promote proliferation of hair follicle stem cells (HFSCs), forcing the hair to start the anagen phase.
Biologic Agents that Promote Hair Growth and Their Mechanisms of Action
SC Signaling
Recently, it has been found that stem cells (SCs) release factors that can promote hair growth. These factors and their mechanisms of action have been summarized in Table 3. These factors, known as “secretomes,” are able to promote skin regeneration, wound healing, and immunologic modulation, among other effects. Some of these factors, such as epidermal growth factor (EGF), basic fibroblast growth factor, hepatocyte growth factor (HGF) and HGF activator, VEGF, insulin-like growth factor (IGF), TGF-ß, and platelet-derived growth factor (PDGF), are able to provide signals that promote hair growth. Dermal papilla cells (DPCs) provide signals to HFSCs located in the bulge that proliferate and migrate either to the dermal papilla (DP) or to the epidermis to repopulate the basal layer.
Enhancement in growth factor expression (except for EGF) has been reported when the adipose SCs are cultured in hypoxic conditions. SCs increase their self-renewal capacity under these conditions. Low oxygen concentrations (1%–5%) increase the level of expression of SC factors that include VEGF, basic fibroblast growth factor, IGF binding protein 1 (IGFBP-1), IGF binding protein 2 (IGFBP-2), macrophage colony-stimulating factor (M-CSF), M-CSF receptor (M-CSFR), and PDGF receptor ß (PDGFR-ß). While these groups of factors promote HF growth in intact skin, another group of factors, such as M-CSF, M-CSFR, and interleukin-6, are involved in wound-induced hair neogenesis. HGF and HGF activator stimulate DPCs to promote proliferation of epithelial follicular cells. EGF promotes cellular migration via the activation of Wnt/ß-catenin signaling. VEGF promotes hair growth and increases the follicle size mainly by perifollicular angiogenesis. Blocking VEGF activity by neutralizing antibodies reduces the size and growth of the HF. PDGF and its receptor (PDGFR-a) are essential for follicular development by promoting upregulation of genes involved in HF differentiation and regulating the anagen phase in HFs. They are also expressed in neonatal skin cells that surround the HF. Monoclonal antibodies to PDGFR-a (APA5) produced failure in hair germ induction, supporting that PDGFR-a and its ligand have an essential role in hair differentiation and development.
IGF-1 promotes proliferation, survival, and migration of HF cells. In addition, IGF binding proteins (IGFBPs) also promote hair growth and hair cell survival by regulating IGF-1 effects and its interaction with extracellular matrix proteins in the HF. Higher levels of IGF-1 and IGFBPs in beard DPCs suggest that IGF-1 levels are associated with androgens. Furthermore, DPCs from non-balding scalps showed significantly higher levels of IGF-1 and IGFBP-6, in contrast to DPCs from balding scalps.
Table 3
Stem Cell Factors and Small Molecules that Promote Hair Growth and Their Mechanisms of Action
Factor | Mechanism of action |
---|---|
HGF and HGF activator | Factor secreted by DPC that promotes proliferation of epithelial follicular cells |
EGF | Promotes growth and migration of follicle ORS cells by activation of Wnt/ß-catenin signaling |
bFGF | Promotes the development of hair follicle |
IL-6 | Involved in WIHN through STAT3 activation |
VEGF | Promotes perifollicular angiogenesis |
TGF-ß | Stimulates the signaling pathways that regulate hair cycle |
IGF-1 | Promotes proliferation, survival, and migration of hair follicle cells |
IGFBP-1 to -6 | Regulates IGF-1 effects and its interaction with extracellular matrix proteins at the hair follicle level |
BMP | Maintains DPC phenotype which is crucial for stimulation of hair follicle stem cell |
BMPR1a | Maintains the proper identity of DPCs that is essential for specific DPC function |
M-CSF | Involved in wound-induced hair regrowth |
M-CSFR | Involved in wound-induced hair regrowth |
PDGF and PDGFR-ß/-a | Upregulates the genes involved in hair follicle differentiation. Induction and regulation of anagen phase. PDGF and its receptors are essential for follicular development |
Wnt3a | Involved in hair follicle development through ß-catenin signaling |
PGE2 | Stimulates anagen phase in hair follicles |
PGF2a and analogs | Promotes transition from telogen to anagen phase of the hair cycle |
BIO | GSK-3 inhibitor |
PGE2 or inhibition of PGD2 or PGD2 receptor D2/GPR44 | Promotes follicle regeneration |
Iron and l-lysine | Under investigation |
Small Molecules
Small molecules with low molecular weight (<900 Da) and the size of 10-9 m are organic compounds that are able to regulate some biologic processes. Some small molecules have been tested for their role in hair growth. Synthetic, non-peptidyl small molecules that act as agonists of the hedgehog pathway have the ability to promote follicular cycling in adult mouse skin. PGE2 and prostaglandin D2 (PGD2) have also been associated with the hair cycle. PGD2 is elevated in the scalp of balding men and inhibits hair lengthening via GPR44 receptor. Also, it is known that PGE2 and PGF2a promote hair growth, while PGD2 inhibits this process. Prostaglandin analogs of PGF2a have been used originally to decrease ocular pressure in glaucoma with parallel effects in the growth of eyelashes, which suggests a specific effect in HF activation. PGD2 receptors are located in the upper and lower ORS region and in the DP, suggesting that these prostaglandins play an important role in hair cycle. Molecules such as quercetin are able to inhibit PGD2 and, in this way, promote hair growth. Antagonists of PGD2 receptor (formally named chemoattractant receptor-homologous expressed in Th2 cells) such as setipiprant have been used to treat allergic diseases such as asthma, but they also have beneficial effects in AGA. Another small molecule l-ascorbic acid 2-phosphate promotes proliferation of ORS keratinocytes through the secretion of IGF-1 from DPCs via phosphatidylinositol 3-kinase. Recently, it has been described that small-molecule inhibitors of Janus kinase–signal transducer and activator of transcription (JAK-STAT) pathway promote hair regrowth in humans. Janus kinase inhibitors are currently approved by the FDA for the treatment of some specific diseases such as psoriasis and other autoimmune-mediated diseases. Also, another group of small molecules such as iron and the amino acid l-Lysine are essential for hair growth.
Cellular Therapy
The multipotent SCs in the bulge region of the HF receive signals from DPCs in order to proliferate and survive. It has been shown that Wnt/ß-catenin signaling is essential for the growth and maintenance of DPCs. These cells can be isolated and cultured in vitro with media supplemented with 10% fetal bovine serum and FGF-2. However, they lose versican expression that correlates with a decrease in follicle-inducing activity in culture. Versican is the most abundant component of HF extracellular matrix. Inhibition of glycogen synthase kinase-3 by (2’Z,3’E)-6-bromoindirubin-3′-oxime (BIO) promotes hair growth in mouse vibrissa follicles in culture by activation of Wnt signaling. Therefore, the increase of Wnt signaling in DPCs apparently is one of the main factors that promote hair growth. DPCs have been also generated from human embryonic SCs that induced HF formation after murine transplantation.
Platelet-Rich Plasma
Platelets are anucleate cells generated by fragmentation of megakaryocytes in the bone marrow. These cells are actively involved in the hemostatic process after releasing biologically active molecules (cytokines). Because of the platelets’ higher capacity to produce and release these factors, autologous platelet-rich plasma (PRP) has been used to treat chronic wounds. Therefore, PRP can be used as autologous therapy for regenerative purposes, for example, chondrogenic differentiation, wound healing, fat grafting, AGA, alopecia areata, facial scars, and dermal volume augmentation. PRP contains human platelets in a small volume that is five to seven times higher than in normal blood and it has been proven to be beneficial to treat AGA. The factors released by these platelets after their activation, such as PDGFs (PDGFaa, PDGFbb, PDGFab), TGF-ß1, TGF-ß2, EGF, VEGF, and FGF, promote proliferation of DPCs and, therefore, may be beneficial for AGA treatment. Clinical experiments indicate that patients with AGA treated with autologous PRP show improved hair count and thickness.
In Search of Novel Therapies
In this paper, we reviewed and discussed the use of therapeutic agents for hair regeneration and the knowledge to promote the development of new therapies for AGA based on the advances in regenerative medicine. The HF is a complex structure that grows when adequate signaling is provided to the HFSCs. These cells are located in the follicle bulge and receive signals from MSCs located in the dermis that are called DPCs. The secretory phenotype of DPCs is determined by local and circulatory signals or hormones. Recent discoveries have demonstrated that SCs in culture are able to activate DPCs and HFSCs and, in this way, promote hair growth. The study of these cellular signals can provide the necessary knowledge for developing more effective therapeutic agents for the treatment of AGA with minimal side effects. Therefore, advancements in the field of regenerative medicine may generate novel therapeutic alternatives. However, further research and clinical studies are needed to evaluate their efficacy.
Disclosure
The authors report no conflicts of interest in this work.
References
1. | Blumeyer A, Tosti A, Messenger A, et al; European Dermatology Forum (EDF). Evidence-based (S3) guideline for the treatment of androgenetic alopecia in women and in men. J Dtsch Dermatol Ges. 2011;9(Suppl 6):S1–S57. | |
2. | Ramos PM, Miot HA. Female pattern hair loss : a clinical and pathophysiological review. An Bras Dermatol. 2015;90(4):529–543. | |
3. | Otberg N, Finner AM, Shapiro J. Androgenetic alopecia. Endocrinolo Metab Clin North Am. 2007;36(2):379–398. | |
4. | Plikus MV, Chuong CM. Complex hair cycle domain patterns and regenerative hair waves in living rodents. J Invest Dermatol. 2008;128(5):1071–1080. | |
5. | McElwee KJ, Shapiro JS. Promising therapies for treating and/or preventing androgenic alopecia. Skin Therapy Lett. 2012;17(6):1–4. | |
6. | Perez-Mora N, Velasco C, Bermüdez F. Oral finasteride presents with sexual-unrelated withdrawal in long-term treated androgenic alopecia in men. Skinmed. 2015;13(3):179–183. | |
7. | Gupta AK, Charrette A. Topical minoxidil: systematic review and meta-analysis of its efficacy in androgenetic alopecia. Skinmed. 2015;13(3):185–189. | |
8. | Shapiro J, Kaufman KD. Use of finasteride in the treatment of men with androgenetic alopecia (male pattern hair loss). J Investig Dermatol Symp Proc. 2003;8(1):20–23. | |
9. | Rossi A, Anzalone A, Fortuna MC, et al. Multi-therapies in androgenetic alopecia: review and clinical experiences. Dermatol Ther. 2016;29(6):424–432. | |
10. | Varothai S, Bergfeld WF. Androgenetic alopecia: an evidence-based treatment update. Am J Clin Dermatol. 2014;15(3):217–230. | |
11. | Rossi A, Cantisani C, Melis L, Iorio A, Scali E, Calvieri S. Minoxidil use in dermatology, side effects and recent patents. Recent Pat Inflamm Allergy Drug Discov. 2012;6(2):130–136. | |
12. | Motofei IG, Rowland DL, Georgescu SR, Mircea T, Baleanu BC, Paunica S. Are hand preference and sexual orientation possible predicting factors for finasteride adverse effects in male androgenic alopecia? Exp Dermatol. 2016;25(7):557–558. | |
13. | Reijo Pera RA, Gleeson JG. Stems cells and regeneration: special review issue. Hum Mol Gen. 2008;17(R1):R1–R2. | |
14. | Jain R, De-Eknamkul W. Potential targets in the discovery of new hair growth promoters for androgenic alopecia. Expert Opin Ther Targets. 2014;18(7):787–806. | |
15. | Park BS, Kim WS, Choi JS, et al. Hair growth stimulated by conditioned medium of adipose-derived stem cells is enhanced by hypoxia : evidence of increased growth factor secretion. Biomed Res. 2010;31(1):27–34. | |
16. | Fukuoka H, Suga H. Hair regeneration treatment using adipose-derived stem cell conditioned medium :follow-up with trichograms. Eplasty. 2015;15:65–72. | |
17. | Du Y, Roh DS, Funderburgh ML, et al. Adipose-derived stem cells differentiate to keratocytes in vitro. Mol Vis. 2010;16:2680–2689. | |
18. | Zhang P, Kling RE, Ravuri SK, et al. A review of adipocyte lineage cells and dermal papilla cells in hair follicle regeneration. J Tissue Eng. 2014;5:2041731414556850. | |
19. | Tsai SY, Sennett R, Rezza A, et al. Wnt/ß-catenin signaling in dermal condensates is required for hair follicle formation. Dev Biol. 2014;385(2):179–188. | |
20. | Choi YS, Zhang Y, Xu M, et al. Distinct functions for Wnt/ß-catenin in hair follicle stem cell proliferation and survival and interfollicular epidermal homeostasis. Cell Stem Cell. 2013;13(6):720–733. | |
21. | Rao TP, Kuhl M. An updated overview on Wnt signaling pathways a prelude for more. Circ Res. 2010;106(12):1798–1806. | |
22. | Yano K, Brown LF, Detmar M. Control of hair growth and follicle size by VEGF-mediated angiogenesis. J Clin Invest. 2001;107(4):409–417. | |
23. | Rendl M, Polak L, Fuchs E. BMP signaling in dermal papilla cells is required for their hair follicle-inductive properties. Genes Dev. 2008;22(4):543–557. | |
24. | Talavera-Adame D, Gupta A, Kurtovic S, Chaiboonma KL, Arumugaswami V, Dafoe DC. Bone morphogenetic protein-2/-4 upregulation promoted by endothelial cells in coculture enhances mouse embryoid body differentiation. Stem Cells Dev. 2013;22(24):3252–3260. | |
25. | Talavera-Adame D, Wu G, He Y, et al. Endothelial Cells in Co-culture Enhance Embryonic Stem Cell Differentiation to Pancreatic Progenitors and Insulin-Producing Cells through BMP Signaling. Stem Cell Rev. 2011;7(3):532–543. | |
26. | Talavera-Adame D, Ng TT, Gupta A, Kurtovic S, Wu GD, Dafoe DC. Characterization of microvascular endothelial cells isolated from the dermis of adult mouse tails. Microvascular Res. 2011;82(2):97–104. | |
27. | Gnedeva K, Vorotelyak E, Cimadamore F, et al. Derivation of hair-inducing cell from human pluripotent stem cells. PLoS One. 2015;10(1):1–14. | |
28. | Lavker RM, Sun T, Oshima H, et al. Hair follicle stem cells. J Investig Dermatol Symp Proc. 2003;8(1):28–38. | |
29. | Bernard B. Advances in understanding hair growth. F1000Res. 2016;5:1–8. | |
30. | Lay K, Kume T, Fuchs E. FOXC1 maintains the hair follicle stem cell niche and governs stem cell quiescence to preserve long-term tissue-regenerating potential. Proc Natl Acad Sci U S A. 2016;113(1): E1506–E1515. | |
31. | Leirós GJ, Attorresi AI, Balañá ME. Hair follicle stem cell differentiation is inhibited through cross-talk between Wnt/ß-catenin and androgen signalling in dermal papilla cells from patients with androgenetic alopecia. Br J Dermatol. 2012;166(5):1035–1042. | |
32. | Chacon-Martinez CA, Klose M, Niemann C, Glauche I, Wickström SA. Hair follicle stem cells= cultures reveal self-organizing plasticity of stem cells and their progeny. EMBO J. 2017;36(2):151–164. | |
33. | Soler AP, Gilliard G, Megosh LC, O’Brien TG. Modulation of murine hair follicle function by alterations in ornithine decarboxylase activity. J Invest Dermatol. 1996;106(5):1108–1113. | |
34. | Hsu YC, Pasolli HA, Fuchs E. Dynamics between stem cells, nich and progeny in the hair follicle. Cell. 2011;144(1):92–105. | |
35. | Kang JI, Kim SC, Kim MK, et al. Effects of dihydrotestosterone on rat dermal papilla cells in vitro. Eur J Pharmacol. 2015;757:74–83. | |
36. | Yamana K, Labrie F, Luu-The V. Human type 3 5a-reductase is expressed in peripheral tissues at higher levels than types 1 and 2 and its activity is potently inhibited by finasteride and dutasteride. Horm Mol Biol Clin Investig. 2010;2(3):293–299. | |
37. | Osada A, Iwabuchi T, Kishimoto J, Hamazaki TS, Okochi H. Long-term culture of mouse vibrissal dermal papilla cells and de novo hair follicle induction. Tissue Eng. 2007;13(5):975–982. | |
38. | Choi GS, Kim JH, Oh SY, et al. Safety and tolerability of the dual 5-alpha reductase inhibitor dutasteride in the treatment of androgenetic alopecia. Ann Dermatol. 2016;28(4):444–450. | |
39. | Carson C 3rd, Rittmaster R. The role of dihydrotestosterone in benign prostatic hyperplasia. Urology. 2003;61(4 Suppl 1):2–7. | |
40. | Kamimura A, Takahashi T. Procyanidin B-2, extracted from apples, promotes hair growth: a laboratory study. Br J Dermatol. 2002;146(1):41–51. | |
41. | Kamimura A, Takahashi T. Procyanidin B-3, isolated from barley and identified as a hair-growth stimulant, has the potential to counteract inhibitory regulation by TGF-beta1. Exp Dermatol. 2002;11(6):532–541. | |
42. | Ulbricht C, Basch E, Bent S, et al. Evidence-based systematic review of saw palmetto by the Natural Standard Research Collaboration. J Soc Integr Oncol. 2006;4(4):170–186. | |
43. | Rondanelli M, Perna S, Peroni G, Guido D. A bibliometric study of scientific literature in Scopus on botanicals for treatment of androgenetic alopecia. J Cosmet Dermatol. 2015;15(2):120–130. | |
44. | Blume-Peytavi U, Kunte C, Krisp A, Garcia Bartels N, Ellwanger U, Hoffmann R. [Comparison of the efficacy and safety of topical minoxidil and topical alfatradiol in the treatment of androgenetic alopecia in women.] J Dtsch Dermatol Ges. 2007;5(5):391–395. German. | |
45. | Farivar S, Malekshahabi T, Shiari R. Biological effects of low level laser therapy. J Lasers Med Sci. 2014;5(2):58–62. | |
46. | Maiman TH. Biomedical lasers evolve toward clinical applications. Hosp Manage. 1966;101(4):39–41. | |
47. | Cung H, Dai T, Sharma SK, et al. The nuts and bolts of low-level laser (light) therapy. Ann Biomed Eng. 2013;40(2):516–533. | |
48. | Bjordal JM, Couppé C, Chow RT, Tunér J, Ljunggren EA. A systematic review of low level laser therapy with location-specific doses for pain from chronic joint disorders. The Aust J Physiother. 2003;49(2):107–116. | |
49. | Brosseau L, Welch V, Wells G, et al. Low level laser therapy (classes I, II and III) in the treatment of rheumatoid arthritis. Cochrane Database Syst Rev. 2005;19(2):CD002049. | |
50. | Cauwels RGEC, Martens LC. Low level laser therapy in oral mucositis: a pilot study. Eur Arch Paediatr Dent. 2011;12(2):118–123. | |
51. | Schindl A, Schindl M, Pernerstorfer-Schön H, Schindl L. Low-intensity laser therapy: a review. J Investig Med. 2000;48(5):312–326. | |
52. | Liu H, Colavitti R, Rovira II, Finkel T. Redox-dependent transcriptional regulation. Cir Res. 2005;97(10):967–974. | |
53. | Gupta AK, Lyons DCA, Abramovits W. Low-level laser/light therapy for androgenetic alopecia. Skinmed. 2014;12(3):145–147. | |
54. | Jimenez JJ, Wikramanayake TC, Bergfeld W, et al. Efficacy and safety of a low-level laser device in the treatment of male and female pattern hair loss: a multicenter, randomized, sham device-controlled, double-blind study. Am J Clin Dermat. 2014;15(2):115–127. | |
55. | Chen CH, Hung HS, Hsu SH. Low-energy laser irradiation increases endothelial cell proliferation, migration, and eNOS gene expression possibly via PI3K signal pathway. Lasers Surg Med. 2008;40(1):46–54. | |
56. | Tuby H, Maltz L, Oron U. Modulations of VEGF and iNOS in the rat heart by low level laser therapy are associated with cardioprotection and enhanced angiogenesis. Lasers Surg Med. 2006;38(7):682–688. | |
57. | Avci P, Gupta GK, Clark J, Wikonkal N, Hamblin MR. Low-level laser (light) therapy (LLLT) for treatment of hair loss. Laser Surg Med. 2015;46(2):144–151. | |
58. | Rodrigues C, de Assis AM, Moura DJ, et al. New therapy of skin repair combining adipose-derived mesenchymal stem cells with sodium carboxymethylcellulose scaffold in a pre-clinical rat model. PloS One. 2014;9(5):e96241. | |
59. | Gimble JM, Katz AJ, Bunnell BA. Adipose-derived stem cells for regenerative medicine. Cir Res. 2007;100(9):1249–1260. | |
60. | Zhang H, Nan W, Wang S, et al. Epidermal growth factor promotes proliferation and migration of follicular outer root sheath cells via Wnt/ß-catenin signaling. Cell Physiol Biochem. 2016;39(1):360–370. | |
61. | Lee YR, Yamazaki M, Mitsui S, Tsuboi R, Ogawa H. Hepatocyte growth factor (HGF) activator expressed in hair follicles is involved in in vitro HGF-dependent hair follicle elongation. J Dermatol Sci. 2001;25(2):156–163. | |
62. | du Cros DL. Fibroblast growth factor influences the development and cycling of murine hair follicles. Dev Biol. 1993;156(2):444–453. | |
63. | Niimori D, Kawano R, Felemban A, et al. Tsukushi controls the hair cycle by regulating TGF-ß1 signaling. Dev Biol. 2012;372(1):81–87. | |
64. | Tomita Y, Akiyama M, Shimizu H. PDGF isoforms induce and maintain anagen phase of murine hair follicles. J Dermatol Sci. 2006;43(2):105–115. | |
65. | Ohyama M, Terunuma A, Tock CL, et al. Characterization and isolation of stem cell – enriched human hair follicle bulge cells. J Clin Invest. 2006;116(1):249–260. | |
66. | Grayson WL, Zhao F, Bunnell B, Ma T. Hypoxia enhances proliferation and tissue formation of human mesenchymal stem cells. Biochem Biophys Res Commun. 2007;358(3):948–953. | |
67. | Potier E, Ferreira E, Andriamanalijaona R, et al. Hypoxia affects mesenchymal stromal cell osteogenic differentiation and angiogenic factor expression. Bone. 2007;40(4):1078–1087. | |
68. | Ren H, Cao Y, Zhao Q, et al. Proliferation and differentiation of bone marrow stromal cells under hypoxic conditions. Biochem Biophys Res Commun. 2006;347(1):12–21. | |
69. | Su HY, Hickford JG, Bickerstaffe R, Palmer BR. Insulin-like growth factor 1 and hair growth. Dermatol Online J. 1999;5(2):1. | |
70. | Batch JA, Mercuri FA, Werther GA. Identification and localization of insulin-like growth factor-binding protein (IGFBP) messenger RNAs in human hair follicle dermal papilla. J Invest Dermatol. 1996;106(3):471–475. | |
71. | Osaka N, Takahashi T, Murakami S, et al. ASK1-dependent recruitment and activation of macrophages induce hair growth in skin wounds. J Cell Biol. 2007;176(7):903–909. | |
72. | Yano K, Brown LF, Detmar M. Control of hair growth and follicle size by VEGF-mediated angiogenesis. J Clin Invest. 2001;107(4):409–417. | |
73. | Takakura N, Yoshida H, Kunisada T, Nishikawa S, Nishikawa SI. Involvement of platelet-derived growth factor receptor-a in hair canal formation. J Invest Dermatol. 1996;107(5):770–777. | |
74. | Panchaprateep R, Asawanonda P. Insulin-like growth factor-1: roles in androgenetic alopecia. Exp Dermatol. 2014;23(3):216–218. | |
75. | Castoreno AB, Eggert US. Small molecule probes of cellular pathways and networks. ACS Chem Biol. 2011;6(1):86–94. | |
76. | Paladini RD, Saleh J, Qian C, Xu GX, Rubin LL. Modulation of hair growth with small molecule agonists of the hedgehog signaling pathway. J Iinvest Dermatol. 2005;125(4):638–646. | |
77. | Nieves A, Garza LA. Does prostaglandin D2 hold the cure to male pattern baldness? Exp Dematol. 2014;(29):224–227. | |
78. | Nelson AM, Loy DE, Lawson JA, Katseff AS, Fitzgerald GA, Garza LA. Prostaglandin D2 inhibits wound-induced hair follicle neogenesis through the receptor Gpr44. J Invest Dermatol. 2013;133(4):881–889. | |
79. | Sasaki S, Hozumi Y, Kondo S. Influence of prostaglandin F2alpha and its analogues on hair regrowth and follicular melanogenesis in a murine model. Exp Dermatol. 2005;14(5):323–328. | |
80. | Choi YM, Diehl J, Levins PC. Promising alternative clinical uses of prostaglandin F2a analogs: beyond the eyelashes. J Am Acad Dermatol. 2015;72(4):712–716. | |
81. | Colombe L, Michelet JF, Bernard BA. Prostanoid receptors in anagen human hair follicles. Exp Dermatol. 2008;17(1):63–72. | |
82. | Zhang Q, Major MB, Takanashi S, et al. Small-molecule synergist of the Wnt/beta-catenin signaling pathway. Proc Natl Acad Sci U S A. 2007;104(18):7444–7448. | |
83. | Fong P, Tong HH, Ng KH, Lao CK, Chong CI, Chao CM. In silicoprediction of prostaglandin D2 synthase inhibitors from herbal constituents for the treatment of hair loss. J Ethnopharmacol. 2015;175:470–480. | |
84. | Weng Z, Zhang B, Asadi S, et al. Quercetin is more effective than cromolyn in blocking human mast cell cytokine release and inhibits contact dermatitis and photosensitivity in humans. PloS One. 2012;7(3):e33805. | |
85. | Norman P. Update on the status of DP2 receptor antagonists; from proof of concept through clinical failures to promising new drugs. Expert Opin Investig Drugs. 2014;23(1):55–66. | |
86. | Pettipher R. The roles of the prostaglandin D(2) receptors DP(1) and CRTH2 in promoting allergic responses. Br J Pharmacol. 2008;153(Suppl 1):S191–S199. | |
87. | Garza LA, Liu Y, Yang Z, et al. Prostaglandin D2 inhibits hair growth and is elevated in bald scalp of men with androgenetic alopecia. Sci Transl Med. 2012;4(126):126ra34. | |
88. | Kwack MH, Shin SH, Kim SR, et al. I-Ascorbic acid 2-phosphate promotes elongation of hair shafts via the secretion of insulin-like growth factor-1 from dermal papilla cells through phosphatidylinositol 3-kinase. Br J Dermatol. 2009;160(6):1157–1162. | |
89. | Harel S, Higgins CA, Cerise JE, et al. Pharmacologic inhibition of JAK-STAT signaling promotes hair growth. Sci Adv. 2015;1(9):e1500973. | |
90. | Quintás-cardama A, Vaddi K, Liu P, et al. INCB018424 : therapeutic implications for the treatment of myeloproliferative neoplasms Preclinical characterization of the selective JAK1 / 2 inhibitor INCB018424 : therapeutic implications for the treatment of myeloproliferative neoplasms. Blood. 2014;115(15):3109–3117. | |
91. | Geyer HL, Mesa RA. Therapy for myeloproliferative neoplasms: when, which agent, and how? Hematology Am Soc Hematol Educ Program. 2014;2014(1):277–286. | |
92. | Ghoreschi K, Jesson MI, Li X, et al. Modulation of innate and adaptive immune responses by tofacitinib (CP-690,550). J Immunol. 2012;186(7):4234–4243. | |
93. | Kontzias A, Laurence A, Gadina M, O’Shea JJ. Kinase inhibitors in the treatment of immune-mediated disease. F1000 Med Rep. 2012;4:5. | |
94. | Bao L, Zhang H, Chan LS. The involvement of the JAK-STAT signaling pathway in chronic inflammatory skin disease atopic dermatitis. JAKSTAT. 2013;2(3):1–8. | |
95. | Rushton DH. Nutritional factors and hair loss. Clin Exp Dermatol. 2002;27(5):396–404. | |
96. | Oshima H, Rochat A, Kedzia C, Kobayashi K, Barrandon Y. Morphogenesis and renewal of hair follicles from adult multipotent stem cells. Cell. 2001;104(2):233–245. | |
97. | Huelsken J, Vogel R, Erdmann B, Cotsarelis G, Birchmeier W. beta-Catenin controls hair follicle morphogenesis and stem cell differentiation in the skin. Cell. 2001;105(4):533–545. | |
98. | Yamauchi K, Kurosaka A. Inhibition of glycogen synthase kinase-3 enhances the expression of alkaline phosphatase and insulin-like growth factor-1 in human primary dermal papilla cell culture and maintains mouse hair bulbs in organ culture. Arch Dermatol Res. 2009;301(5):357–365. | |
99. | Sotoodehnejadnematalahi F, Burke B. Structure, function and regulation of versican: the most abundant type of proteoglycan in the extracellular matrix. Acta Med Iran. 2013;51(11):740–750. | |
100. | Xu XR, Zhang D, Oswald BE, et al. Platelets are versatile cells: new discoveries in hemostasis, thrombosis, immune responses, tumor metastasis and beyond. Crit Rev Clin Lab Sci. 2016;53(6):409–430. | |
101. | Lacci KM, Dardik A. Platelet-rich plasma: support for its use in wound healing. Yale J Biol Med. 2010;83(1):1–9. | |
102. | Mussano F, Genova T, Munaron L, Petrillo S, Erovigni F, Carossa S. Cytokine, chemokine, and growth factor profile of platelet-rich plasma. Platelets. 2016;27(5):467–471. | |
103. | Martinez-Zapata MJ, Martí-Carvajal AJ, Solà I, et al. Autologous platelet-rich plasma for treating chronic wounds. Cochrane Database Syst Rev. 2016;(5):CD006899. | |
104. | Modarressi A. Platlet Rich Plasma (PRP) improves fat grafting outcomes. World J Plast Surg. 2013;2(1):6–13. | |
105. | Gentile P, Garcovich S, Bielli A, Scioli MG, Orlandi A, Cervelli V. The Effect of platelet-rich plasma in hair regrowth: a randomized placebo-controlled trial. Stem Cells Transl Med. 2015;4(11):1317–1323. | |
106. | Lynch MD, Bashir S. Applications of platelet-rich plasma in dermatology: a critical appraisal of the literature. J Dermatolog Treat. 2016;27(3):285–289. | |
107. | Trink A, Sorbellini E, Bezzola P, et al. A randomized, double-blind, placebo- and active-controlled, half-head study to evaluate the effects of platelet-rich plasma on alopecia areata. Br J Dermatol. 2013;169(3):690–694. | |
108. | Oh Y, Kim BJ, Kim MN. Depressed facial scars successfully treated with autologous platelet-rich plasma and light-emitting diode phototherapy at 830 nm. Ann Dermatol. 2014;26(3):417–418. | |
109. | Singhal P, Agarwal S, Dhot PS, Sayal SK. Efficacy of platelet-rich plasma in treatment of androgenic alopecia. Asian J Transfus Sci. 2015;9(2):159–162. | |
110. | Valente Duarte de Sousa IC, Tosti A. New investigational drugs for androgenetic alopecia. Expert Opin Investig Drugs. 2013;22(5):573–589. | |
111. | Alves R, Grimalt R. Randomized placebo-controlled, double-blind, half-head study to assess the efficacy of platelet-rich plasma on the treatment of androgenetic alopecia. Dermatol Surg. 2016;42(4):491–497. | |
112. | Cho JW, Kim SA, Lee KS. Platelet-rich plasma induces increased expression of G1 cell cycle regulators, type I collagen, and matrix metalloproteinase-1 in human skin fibroblasts. Int J Mol Med. 2012;29(1):32–36. | |
113. | Mehta V. Platelet-rich plasma: a review of the science and possible clinical applications. Orthopedics. 2010;33(2):111. | |
114. | Leo MS, Kumar AS, Kirit R, Konathan R, Sivamani RK. Systematic review of the use of platelet-rich plasma in aesthetic dermatology. J Cosmet Dermatol. 2015;14(4):315–323. |
- Published in Corporate News / Blog
Phase I/II Study of Safety and Preliminary Efficacy of Intravenous Allogeneic Mesenchymal Stem Cells in Chronic Stroke
Authors:
Michael L. Levy, MD, PhD; John R. Crawford, MD; Nabil Dib, MD; Lev Verkh, PhD; Nikolai Tankovich, MD, PhD; Steven C. Cramer, MD
Background and Purpose
Stroke is a leading cause of long-term disability. Limited treatment options exist for patients with chronic stroke and substantial functional deficits. This study examined the safety and preliminary efficacy of intravenous allogeneic mesenchymal stem cells in this population.
Methods
Entry Criteria
- Ischemic stroke >6 months prior
- Substantial impairment (National Institutes of Health Stroke Scale score ≥6)
- Disability
Treatment Procedure
- Enrollees received a single intravenous dose of allogeneic ischemia-tolerant mesenchymal stem cells.
- Phase 1 used a dose-escalation design (3 tiers, n=5 each).
- Phase 2 was an expanded safety cohort.
Primary and Secondary End Points
- The primary end point was safety over 1 year.
- Secondary end points examined behavioral change.
Results
Phase 1 Findings (n=15)
- Each dose (0.5, 1.0, and 1.5 million cells/kg body weight) was found safe.
Phase 2 Findings (n=21)
- Subjects received 1.5 million cells/kg.
- At baseline, subjects (n=36) averaged 4.2±4.6 years post-stroke, age 61.1±10.8 years, National Institutes of Health Stroke Scale score 8 (6.5–10), and Barthel Index 65±29.
- Two were lost to follow-up, one was withdrawn, and two died (unrelated to study treatment).
Adverse Events
- Of 15 serious adverse events, none was possibly or probably related to study treatment.
- Two mild adverse events were possibly related to study treatment: a urinary tract infection and intravenous site irritation.
- Treatment was safe based on serial exams, electrocardiograms, laboratory tests, and computed tomography scans of chest/abdomen/pelvis.
Behavioral End Points
- All behavioral end points showed significant gains over the 12 months of follow-up.
- Barthel Index scores increased by 6.8±11.4 points at 6 months (P=0.002) and by 10.8±15.5 points at 12 months (P<0.001) post-infusion.
- The proportion of patients achieving excellent functional outcomes (Barthel score ≥95) increased from 11.4% at baseline to 27.3% at 6 months and to 35.5% at 12 months.
Conclusions
Intravenous transfusion of allogeneic ischemia-tolerant mesenchymal stem cells in patients with chronic stroke and substantial functional deficits was safe and suggested behavioral gains. These data support proceeding to a randomized, placebo-controlled study of this therapy in this population.
Clinical Trial Registration
URL: http://www.clinicaltrials.gov
Unique identifier: NCT01297413
Key Words: abdomen, brain ischemia, neuroprotection, pelvis, reperfusion
Mesenchymal Stem Cells as Restorative Therapy for Stroke Patients
Stroke is perennially among the leading causes of human disability and the leading neurological cause of lost disability-adjusted life years. The mean survival after stroke is 6 to 7 years, and indeed more than 85% of patients live past the first year poststroke, many with years of enduring disability. Many restorative therapies are under study to improve outcomes after stroke. Restorative therapies aim to improve patient outcomes by promoting the neural processes underlying behavioral recovery and are distinguished from acute therapies, such as reperfusion or neuroprotection, that aim to reduce initial injury. As such, restorative therapies often have a time window measured in days to months, or in some cases, years.
Mesenchymal Stem Cells (MSC) as a Candidate for Stroke Therapy
Mesenchymal stem cells (MSC), also known as mesenchymal stromal cells, are among the leading restorative therapy candidates. Substantial preclinical data support the safety and efficacy of MSC as a restorative therapy to improve outcomes after stroke. For example, a meta-analysis reported that 44 of 46 preclinical stroke studies found MSC to be superior to placebo, with effect sizes greater than 1.0.
Initial Human Studies of MSC
Initial human studies of MSC (or MSC-like cells) after stroke focused on autologous cell therapies, whereby bone marrow is taken from each patient to produce his/her own MSC batch, and found MSC infusion to be safe. MSC are relatively immunoprivileged given their very low levels of human leukocyte antigen molecule expression, a fact that opens the door to the administration of allogeneic MSC. Allogeneic MSC have been found to be safe without the use of concomitant immunosuppression, and can be manufactured in a manner that enables broad clinical application.
Studies of Allogeneic MSC
Studies of allogeneic MSC (or MSC-like cells) poststroke have focused on early time points (administration 24–48 hours poststroke) or used an invasive procedure to implant cells intracerebrally. Each approach has its relative advantages and disadvantages, and an intravenous method of introducing MSC, if comparably efficacious, might facilitate widespread implementation and also avoid adverse events attributable to invasive procedures.
Current Study Overview
The current study was a phase I/II dose-escalation trial that examined the effects of a single intravenous infusion of allogeneic ischemia-tolerant MSC. The target population was patients with chronic ischemic stroke and substantial functional deficits, a group for whom treatment options remain limited. The primary outcome was safety, based on serial measures of behavior, computed tomography (CT) scans, and laboratory testing. Preliminary estimates of treatment efficacy were also examined.
Inclusion Criteria
- Age ≥18 years
- Ischemic stroke ≥6 months prior, radiologically confirmed at initial diagnosis and at study enrollment
- Severe disability resulting from the index stroke, operationally defined as subject confined to a wheelchair, requiring home nursing care, or needing assistance with activities of daily living
- No substantial improvement in neurological or functional status for the 2 months before study enrollment
- NIHSS score 6–20
- Life expectancy >12 months
- Patient receiving standard of care secondary stroke prevention before enrollment
- Patient or a surrogate able to provide informed consent
- Reasonable expectation that the patient will receive standard posttreatment care and attend all scheduled study visits
- Adequate systemic organ function, specifically:
- Serum aspartate aminotransferase ≤2.5× upper limit of normal
- Serum alanine aminotransferase ≤2.5× upper limit of normal
- Total serum bilirubin ≤1.5× upper limit of normal
- Prothrombin time and partial thromboplastin time ≤1.25× upper limit of normal in subjects not receiving antithrombotic therapy
- Serum albumin ≥3.0 g/dL
- Absolute neutrophil count ≥1500/µL
- Platelet count ≥150,000/µL
- Hemoglobin ≥9.0 g/dL
- Serum creatinine ≤1.5× upper limit of normal
- Serum amylase or lipase ≤1.0× upper limit of normal
Exclusion Criteria
- History of uncontrolled seizure disorder
- History of cancer within the past 5 years, except for localized basal or squamous cell carcinoma
- History of cerebral neoplasm
- Positive for hepatitis B, C, or HIV
- Myocardial infarction within 6 months of study entry
- Presence of any other clinically significant medical or psychiatric condition, or laboratory abnormality, for which study participation would pose a safety risk in the judgment of the Investigator or Sponsor
- Findings on baseline computed tomography suggestive of subarachnoid or intracerebral hemorrhage within the past 12 months
- Participation in another investigational drug or device study in the 3 months before treatment
- History within the past year of drug or alcohol abuse
- Pregnant or lactating, or expectation to become pregnant during the study
- Allergy to bovine or porcine products
NIHSS indicates National Institutes of Health Stroke Scale.
Methods
Study Design
This was a phase I/II multi-center, open-label study that aimed to evaluate the safety and preliminary efficacy of a single intravenous infusion of marrow-derived allogeneic ischemia-tolerant MSC. Entry criteria appear in Table 1 and in sum describe enrollment of adults with radiologically verified chronic stable ischemic stroke and substantial impairment and functional deficits. Patients were followed for one year after MSC infusion. The study made no restrictions on, and did not provide any forms of, medication or therapy (occupational, physical, or speech) during the follow-up year after infusion. All patients signed consent in accordance with local Institutional Review Board approval. This study was approved by the Food and Drug Administration and was registered at clinicaltrials.gov. The data that support the findings of this study are available from the corresponding author on reasonable request.
The study occurred in 2 parts, with part 1 being a dose-escalation study and part 2 being an expanded safety study based on part 1 findings. Part 1 consisted of 3 cohorts (n=5 per cohort) enrolled sequentially in a dose-escalation manner, with subjects receiving one of 3 doses based on body weight, with a maximum dosage of 150 million cells. Cohort 1 received 0.5 million cells/kg of body weight; Cohort 2, 1.0 million cells/kg; and Cohort 3, 1.5 million cells/kg. The dose-escalation plan in part 1 required a review by the Data Safety Monitoring Board once the 5 subjects in Cohort 1 were treated and evaluated through study day 10. If safety was established, Cohort 2 was to proceed at the next highest dose, followed by a similar safety review before escalation to the highest dose in Cohort 3. Part 2 aimed to enroll an additional minimum of 20 subjects at the highest safe dose level determined in part 1. An additional interim review was conducted by the Data Safety Monitoring Board after the first 5 patients were treated in part 2. Detailed stopping rules appear in the online-only Data Supplement (see Stopping Rules and Determination of Maximum Tolerated Dose).
The target dose of 1.5 million cells/kg corresponds to allometric scaling from animal studies. Our meta-analysis of preclinical studies of MSC after experimental ischemic stroke identified 9 rodent studies that transfused MSC using the intravenous route in the post-acute period. In each study, MSC provided substantial behavioral gains (effect sizes >1.0), using doses ranging from 3.6 to 12.4×10^6 MSC/kg body weight (mean dose of 10.1×10^6 MSC/kg). The approach to allometric scaling from animals to humans recommended by the Food and Drug Administration uses a body surface area normalization, which for the mean value in rodents yields a comparable human dose of 1.6×10^6 MSC/kg.
Cell Manufacturing and Shipping
Manufacturing of MSC was performed at the GMP-compliant facility of the sponsor, Stemedica Cell Technologies, Inc (San Diego, CA). MSC were grown from the bone marrow of a single human donor and are from the same batch used in prior preclinical and clinical studies. Cells were grown under low oxygen (5%) conditions. Such ischemia-tolerant MSC have advantages compared with those grown under normoxic conditions, for example, showing higher proliferation rate, expression of stem cell-related genes, production of key cytokines, and migration activity. Cells were harvested at passage 4 and expressed CD105, CD73, and CD90 surface markers, consistent with the International Society for Cellular Therapy definition. Cells were cryopreserved by suspending in Cryostar CS10 freezing medium (BioLife Solutions, Bothell, WA) then stored in the vapor phase of liquid nitrogen. This parent cell bank was then tested for quality control including cell count, viability, appearance, and quantitative polymerase chain reaction for viruses including HIV, Epstein-Barr virus, cytomegalovirus, hepatitis B virus, parvovirus B19, and hepatitis C virus. Cryovials were shipped at ≤-150°C in a vapor phase liquid nitrogen shipper with temperature monitor.
Infusion of Investigational Product
Each site’s pharmacy prepared MSC for infusion per a study-provided protocol. Cryovials (the number of which was based on the dose to be infused) were thawed and MSC were washed in, and then suspended in, Lactated Ringer’s solution at a concentration of 1×10^6 cells/mL using one to three 60 mL syringes. The suspension then underwent final testing before being released for intravenous infusion, consisting of cell count, endotoxin, Gram stain, and review of appearance. Cell count was performed using 0.1% Trypan Blue and a hemacytometer, which also yielded % cell viability. The minimum percent cell viability was required to be ≥70% for the cells to be released. A sample was also sent for subsequent sterility testing. After release by the pharmacy, the final formulation was stored at 2° to 8°C and infused within 8 hours of preparation.
MSC Administration
Before MSC infusion, a 0.1 mL aliquot of the final MSC formulation was injected intradermally; any subject showing a positive reaction (e.g., wheal with erythema) would not be infused. Cells were administered intravenously via metered-dose syringe pump at 2 mL/min. Patients remained in the inpatient telemetry unit for observation until clinically stable.
Patient Assessments
Patients had frequent monitoring until discharged from the telemetry unit. After discharge, patients had safety evaluations on day 2, 3, 4, and 10, then again on month 1, 3, 6, 9, and 12. Adverse events were coded according to the MedDRA adverse event dictionary. The relationship that adverse events had to the investigational product was assessed by the site investigator. Patients were followed for one year using tests of behavior, serology, blood chemistry and cell counts, electrocardiogram, urine, and CT of chest, abdomen, and pelvis. The full schedule of assessments appears in Table SI in the online-only Data Supplement.
Statistics
The primary study endpoint was safety and tolerability, evaluated in all subjects who received any portion of an infusion, and determined by the incidence/severity of adverse events, clinically significant changes on laboratory and imaging tests, vital signs, and physical plus neurological examinations. Four secondary endpoints were scored serially to derive preliminary estimates of efficacy: National Institutes of Health Stroke Scale, Barthel Index (BI), Mini-Mental Status Exam, and Geriatric Depression Scale. For each, the change from baseline was evaluated using the Wilcoxon signed-rank test, with primary analysis of preliminary efficacy being change from baseline to 6 months post-infusion, and analysis including all subjects who received an infusion except for one subject who failed to return after the day 10 visit for all visits (except for month 9 follow-up). For any subject missing 6-month data, 9-month or 12-month data were substituted for this analysis, otherwise missing data were not imputed. Data were analyzed using R statistical software. Given the exploratory nature of this study, sample size was selected as appropriate for detection of any safety concerns in an early phase clinical trial.
Results
Subjects
Of 50 subjects who seemed eligible on prescreening, 36 were enrolled and received treatment from March 14, 2011, to December 15, 2016 (Figure and Table 2). There were 13 subjects enrolled at the University of California, San Diego, 19 subjects at Arizona, and 4 subjects at the University of California, Irvine. Interim safety reviews disclosed no concerns, and so 5 subjects received 0.5×10^6 cells/kg in part 1/Cohort 1, 5 subjects received 1.0×10^6 cells/kg in part 1/Cohort 2, 5 subjects received 1.5×10^6 cells/kg in part 1/Cohort 3, and all 21 subjects in part 2 received 1.5×10^6 cells/kg. For the 15 subjects in part 1, 12 completed the study, 2 died of unrelated causes (coronary artery disease 6 months post-infusion and sepsis 1 month after infusion), and 1 was lost to follow-up after day 10 (reappearing only for the month 9 follow-up visit). For the 21 subjects in part 2, 19 completed the study, 1 was lost to follow-up after month 6, and 1 was withdrawn by the site PI after month 6 due to treatment with another investigational product. Of the 36 subjects enrolled, the planned dose was delivered within 2 mL (i.e., within 2×10^6 cells) of the target in 26 subjects, whereas in 10 subjects a median of 7.6 (interquartile range, 4.4–10.25) mL (i.e., 7.6×10^6 cells) was not infused as planned, which represented a median of 6.5% (5.3–9.8) of the intended dose. A total of 179 protocol deviations were reported, mainly related to scheduling study visits or study testing (Table SII in the online-only Data Supplement).
Figure. CONSORT diagram.
Table 2. Baseline Subject Characteristics
Characteristic | Cohort 1 | Cohort 2 | Cohort 3 | Total |
---|---|---|---|---|
n | 5 | 5 | 5 | 21 |
Sex | ||||
– Male | 5 (100%) | 4 (80%) | 4 (80%) | 14 (66.67%) |
– Female | 0 (0%) | 1 (20%) | 1 (20%) | 7 (33.33%) |
Age, y | 50.8 ± 9.8 | 56.8 ± 11.1 | 68.8 ± 11.58 | 62.8 ± 9.2 |
[40—62] | [39—69] | [53—84] | [51—83] | |
Race | ||||
– White | 4 (80%) | 3 (60%) | 5 (100%) | 17 (80.95%) |
– Asian | 0 (0%) | 1 (20%) | 0 (0%) | 0 (0%) |
– American Indian/Alaskan Native | 0 (0%) | 1 (20%) | 0 (0%) | 0 (0%) |
– Native Hawaiian/Pacific Islander | 0 (0%) | 0 (0%) | 0 (0%) | 0 (0%) |
– Black | 1 (20%) | 0 (0%) | 0 (0%) | 1 (4.76%) |
– Other | 0 (0%) | 0 (0%) | 0 (0%) | 3 (14.29%) |
Ethnicity | ||||
– Hispanic or Latino | 0 (0%) | 0 (0%) | 0 (0%) | 2 (9.52%) |
– Non-Hispanic or Non-Latino | 5 (100%) | 5 (100%) | 5 (100%) | 19 (90.48%) |
Living situation | ||||
– At home | 5 (100%) | 5 (100%) | 3 (60%) | 19 (90.48%) |
– In a living facility | 0 (0%) | 0 (0%) | 2 (40%) | 2 (9.52%) |
Time from stroke to infusion, y | 1.6 ± 0.9 | 7.7 ± 5.0 | 4.1 ± 2.2 | 4.0 ± 5.0 |
[0.6—2.9] | [1.1—14.5] | [1.7—7.0] | [0.7—24.8] | |
Total | 5 | 5 | 5 | 36 |
Safety
A total of 15 serious adverse events were reported. These were wide-ranging in nature, for example, infections, vascular disorders, and pain syndromes (for full details, see Table SIII in the online-only Data Supplement). All serious adverse events were deemed unrelated or unlikely related to the investigational product. A total of 109 adverse events were reported, of which 2, both mild, were considered by the site investigator to be possibly related to the investigational product: one urinary tract infection and one report of intravenous site irritation. Both adverse events recovered completely.
Study testing disclosed no safety concerns. No subject showed a preinfusion positive reaction to intradermal testing. Serial physical exams and blood testing did not disclose any significant findings. Only one of the serial electrocardiograms was thought to have clinically significant findings, in a subject with moderate intraventricular conduction delay, only at the 1-month follow-up visit. Similarly, across serial CT scans of the chest, abdomen, and pelvis, only one was considered clinically significant, a soft tissue density in the anterior abdominal wall seen at 6-months that was stable when reimaged at 12-months.
Behavioral Effects
Across all subjects, improvements were seen in National Institutes of Health Stroke Scale, BI, Mini-Mental Status Exam, and Geriatric Depression Scale scores at both the 6-month and the 12-month follow-up visits (Table 3). These were statistically significant, generally stable over time, and clinically modest in magnitude. Most findings would survive correction for multiple comparisons. Changes in the BI suggest clinical utility, with a 6.8 point gain by 6-months that grew to a 10.8 point gain by 12-months post-infusion (P<0.001), and with the proportion of patients achieving excellent functional outcome (Barthel score =95) increasing from 11.4% (4/35) at baseline to 9/33 (27.3%) at 6-months to 35.5% (11/31) at 12-months.
Discussion
Stroke is a major cause of human disability. This can be reduced by acute therapies that are introduced in the early hours poststroke to reduce initial injury, and by restorative therapies that are introduced days, months, or years poststroke to promote neural repair. Allogeneic MSC show substantial favorable effects in preclinical studies, including when introduced via the intravenous route. The current study found a single intravenous infusion of allogeneic MSC to be safe and potentially associated with functional improvement.
The current study is the largest trial of intravenous MSC in patients with chronic stroke and the first to evaluate allogeneic MSC therapy in this population. It is also the first human stroke study to evaluate MSC grown under hypoxic conditions, which favorably affects cell proliferation, gene expression, cytokine production, and migration. Intravenous infusion of MSC was found to be safe in 36 patients who had chronic stroke with substantial functional deficits. Across 3 escalating doses, treatment-related adverse events were infrequent, mild, and transient. Serial assessments of exam, laboratory testing, electrocardiogram, and CT scans of chest/abdomen/pelvis disclosed no safety concerns, with limited subject dropout. These results are consistent with the overall excellent safety record that MSC have in clinical trials of human subjects across numerous non-cerebrovascular diagnoses and in stroke trials.
Patients with stroke in the chronic stage generally show functional decline; however, enrollees in the current study showed 12 months of continued functional improvement. In general, recovery from stroke-related deficits shows a bimodal time course. Initially, most stroke survivors show some degree of spontaneous recovery, for example, during the initial months for the motor system. Within a year of stroke onset, however, a significant decline in function is commonly seen. This is significant given that few treatment options are available to improve function in patients in the chronic phase of stroke. In the current study, behavioral gains were seen, though were modest in magnitude. However, a 2-point improvement in the National Institutes of Health Stroke Scale score in the setting of chronic stroke, if verified in a larger controlled study, might be regarded as important. Also, the mean gain in BI from baseline grew to 10.8 points by 12 month-poststroke (P<0.001), higher than the BI minimal clinically important difference of 9.25 points. Furthermore, the proportion of patients with an excellent functional outcome (BI score =95) increased from 11.4% at baseline to 27.3% at 6-months and to 35.5% at 12-months. This 12-month period of continued functional improvement is consistent with preclinical studies examining the distribution of systemically administered MSC: intravenous MSC given early after stroke initially localize to lungs then spleen, then increase within the region of brain ischemia, and by 30 days poststroke are concentrated in the peri-infarct region. At one year, most surviving MSC are in the peri-infarct region, with very few present in other organs. Patients also showed significant improvement in the Mini-Mental Status Exam and Geriatric Depression Scale, changes that were largely sustained at 12 months post-infusion, suggesting that MSC have broad effects on brain function. These findings require verification in a larger, controlled study but raise hope that this intervention could improve functional status in the chronic stroke setting. Future studies might also incorporate modality-specific outcome measures to provide more granular assessments of behavioral gains in individual neural systems.
Table 3. Behavioral Change Over Time
Measure | Baseline | Change to 6 mo | Change to 12 mo |
---|---|---|---|
Mini-Mental Status Exam score | 24.2±6.0 (n=35) | 1.8±2.8 (n=32) | 1.3±2.7 (n=31) |
P Value | <0.001 | 0.017 | |
NIHSS score | 8 [6.5 to 10] | -1 [-2.25 to 0] | -2 [-3.5 to -0.5] |
P Value | <0.001 | <0.001 | |
Geriatric depression scale score | 5.1±3.5 (n=35) | -1.6±3.8 (n=32) | -1.4±3.8 (n=31) |
P Value | 0.015 | ||
Barthel Index (score) | 65±28.7 (n=35) | 6.8±11.4 (n=33) | 10.8±15.5 (n=31) |
P Value | 0.002 | <0.001 | |
Barthel Index (% =95) | |||
Proportion at baseline | 4 (11.4%) | 9 (27.3%) | 11 (35.5%) |
P Value | 0.015 | 0.01 |
Meta-analysis of MSC Effects in Animals with Experimental Ischemic Stroke
Meta-analysis conducted on MSC effects in animals with experimental ischemic stroke demonstrated substantial and sustained effect sizes, even after adjusting for potential publication bias. This effect was consistent across various factors such as species, delivery route, timing of administration post-stroke, and dosage. Preclinical studies have shown that MSC introduction up to 1 month or 4 to 6 weeks post-infarct yields promising results.
Bidirectional Translation: Bedside Experience Informing Preclinical Studies
Findings from patients many months post-stroke (refer to Table 2) underscore the necessity for bidirectional translation. This approach involves translating bedside experiences into insights that inform and refine preclinical studies. This iterative process enhances the applicability and efficacy of future treatments.
Study Strengths
Population with Substantial Functional Deficits
The study enrolled patients in the chronic stage of stroke, a demographic numbering in the millions worldwide, with limited treatment options.
Utilization of Allogeneic MSC
The study utilized allogeneic MSC due to their relatively immunoprivileged nature, eliminating the need for immunosuppression. This approach enables broader implementation within the stroke population.
Safety Evaluation
A dose-escalation design evaluated safety aspects rigorously. Cell culture was limited to 4 passages to optimize MSC features crucial for efficacy.
Study Limitations
Lack of Control Group
Since the study focused primarily on safety, the absence of a control group complicates the interpretation of observed behavioral improvements (refer to Table 3).
Mechanism of Action
The mechanism of action underlying cell therapies improving outcomes in the chronic phase remains unexplored. Future trials should investigate mechanisms like growth factor release, anti-inflammatory effects, and exosome involvement.
Conclusion: Implications for Future Research
This study establishes the safety of intravenous allogeneic MSC in chronic stroke patients with substantial functional deficits. While suggesting functional benefits, further verification through controlled studies is imperative. These findings advocate for continued research into intravenous allogeneic MSC for chronic stroke, including mechanistic exploration.
To improve the on-page SEO for your article and add H2 headings for references, here’s a structured approach:
References
- Feigin VL, Lawes CM, Bennett DA, Anderson CS. Stroke epidemiology: a review of population-based studies of incidence, prevalence, and case-fatality in the late 20th century. Lancet Neurol. 2003;2:43–53.
- Johnston SC, Hauser SL. Neurological disease on the global agenda. Ann Neurol. 2008;64:A11–A12.
- Lloyd-Jones D, Adams RJ, Brown TM, et al. Heart disease and stroke statistics–2010 update: a report from the American Heart Association. Circulation. 2010;121:e46–e215.
- Lin DJ, Finklestein SP, Cramer SC. New directions in treatments targeting stroke recovery. Stroke. 2018;49:3107–3114. doi: 10.1161/STROKEAHA.118.021359
- Cramer SC. Repairing the human brain after stroke. II. Restorative therapies. Ann Neurol. 2008;63:549–560. doi: 10.1002/ana.21412
- Wolf SL, Winstein CJ, Miller JP, et al; EXCITE Investigators. Effect of constraint-induced movement therapy on upper extremity function 3 to 9 months after stroke: the EXCITE randomized clinical trial. JAMA. 2006;296:2095–2104. doi: 10.1001/jama.296.17.2095
- McCabe J, Monkiewicz M, Holcomb J, et al. Comparison of robotics, functional electrical stimulation, and motor learning methods for treatment of persistent upper extremity dysfunction after stroke: a randomized controlled trial. Arch Phys Med Rehabil. 2015;96:981–990. doi: 10.1016/j.apmr.2014.10.022
- Dodakian L, McKenzie AL, Le V, et al. A home-based telerehabilitation program for patients with stroke. Neurorehabil Neural Repair. 2017;31:923–933. doi: 10.1177/1545968317733818
- Ward NS, Brander F, Kelly K. Intensive upper limb neurorehabilitation in chronic stroke: outcomes from the Queen Square programme. J Neurol Neurosurg Psychiatry. 2019;90:498–506. doi: 10.1136/jnnp-2018-319954
- Vu Q, Xie K, Eckert M, et al. Meta-analysis of preclinical studies of mesenchymal stromal cells for ischemic stroke. Neurology. 2014;82:1277–1286. doi: 10.1212/WNL.0000000000000278
- Bang OY, Lee JS, Lee PH, Lee G. Autologous mesenchymal stem cell transplantation in stroke patients. Ann Neurol. 2005;57:874–882. doi: 10.1002/ana.20501
- Honmou O, Houkin K, Matsunaga T, et al. Intravenous administration of auto serum-expanded autologous mesenchymal stem cells in stroke. Brain. 2011;134(pt 6):1790–1807. doi: 10.1093/brain/awr063
- Bhasin A, Srivastava MV, Kumaran SS, et al. Autologous mesenchymal stem cells in chronic stroke. Cerebrovasc Dis Extra. 2011;1:93–104. doi: 10.1159/000333381
- Le Blanc K, Tammik C, Rosendahl K, et al. HLA expression and immunologic properties of differentiated and undifferentiated mesenchymal stem cells. Exp Hematol. 2003;31:890–896.
- Lalu MM, McIntyre L, Pugliese C, et al; Canadian Critical Care Trials Group. Safety of cell therapy with mesenchymal stromal cells (SafeCell): a systematic review and meta-analysis of clinical trials. PLoS One. 2012;7:e47559. doi: 10.1371/journal.pone.0047559
- Hess DC, Wechsler LR, Clark WM, et al. Safety and efficacy of multipotent adult progenitor cells in acute ischemic stroke (MASTERS): a randomized, double-blind, placebo-controlled, phase 2 trial. Lancet Neurol. 2017;16:360–368. doi: 10.1016/S1474-4422(17)30046-7
- Steinberg GK, Kondziolka D, Wechsler LR, et al. Clinical outcomes of transplanted modified bone marrow-derived mesenchymal stem cells in stroke: a phase 1/2a study. Stroke. 2016;47:1817–1824. doi: 10.1161/STROKEAHA.116.012995
- Guidance for Industry: Estimating the Maximum Safe Starting Dose in Initial Clinical Trials for Therapeutics in Adult Healthy Volunteers. 2005. www.fda.gov/downloads/Drugs/GuidanceComplianceRegulatoryInformation/Guidances/ucm078932.pdf. Accessed June 22, 2019.
- Luger D, Lipinski MJ, Westman PC, et al. Intravenously delivered mesenchymal stem cells: systemic anti-inflammatory effects improve left ventricular dysfunction in acute myocardial infarction and ischemic cardiomyopathy. Circ Res. 2017;120:1598–1613. doi: 10.1161/CIRCRESAHA.117.310599
- Harach T, Jammes F, Muller C, et al. Administrations of human adult ischemia-tolerant mesenchymal stem cells and factors reduce amyloid beta pathology in a mouse model of Alzheimer’s disease. Neurobiol Aging. 2017;51:83–96. doi: 10.1016/j.neurobiolaging.2016.11.009
- Butler J, Epstein SE, Greene SJ, et al. Intravenous allogeneic mesenchymal stem cells for nonischemic cardiomyopathy: safety and efficacy results of a phase II-a randomized trial. Circ Res. 2017;120:332–340. doi: 10.1161/CIRCRESAHA.116.309717
- Vertelov G, Kharazi L, Muralidhar MG, et al. High targeted migration of human mesenchymal stem cells grown in hypoxia is associated with enhanced activation of RhoA. Stem Cell Res Ther. 2013;4:5. doi: 10.1186/scrt153
- Dominici M, Le Blanc K, Mueller I, et al. Minimal criteria for defining multipotent mesenchymal stromal cells. The International Society for Cellular Therapy position statement. Cytotherapy. 2006;8:315–317. doi: 10.1080/14653240600855905
- Devine SM. Mesenchymal stem cells: will they have a role in the clinic? J Cell Biochem Suppl. 2002;38:73–79.
- Hilfiker A, Kasper C, Hass R, Haverich A. Mesenchymal stem cells and progenitor cells in connective tissue engineering and regenerative medicine: is there a future for transplantation? Langenbecks Arch Surg. 2011;396:489–497. doi: 10.1007/s00423-011-0762-
- Published in Corporate News / Blog